Ce travail a été réalisé en collaboration avec l'IRSN/DPAM (Institut de Radioprotection et de Sûreté Nucléaire/Direction de Prévention des Accidents Majeurs de Cadarache) que je remercie de m'avoir choisi comme candidat pour la réalisation de cette recherche. travail. Ces outils de simulation sont développés au département de modélisation DPAM de l'IRSN pour analyser le comportement de l'iode lors de son transfert entre le cœur fondu du réacteur et l'enceinte de confinement.
CONTEXTE: L’EVALUATION DU TERME SOURCE IODE EN CAS D’ACCIDENT
- Accident grave de réacteur nucléaire
- Objectif du programme CHIP
- Spécification des conditions aux limites pour la réalisation des essais analytique 18
Determination of the appearance potentials of each ion (for the parent ion this is the ionization potential). Logarithms of the product IT (proportional to the partial pressure) as a function of the.

SPECTROMETRIE DE MASSE À HAUTE TEMPERATURE
- Principe
- Les cellules d’effusions couplées au SMHT
- Etalonnage/Calibration du spectromètre
- Description du spectromètre
- Gamme de mesure spectrométrique
MÉTHODE D’INTERPRÉTATION: 2IÈME ET 3IÈME LOI DE LA
ROPRIÉTÉS THERMODYNAMIQUES DU SYSTÈME CS-I-O-H
- Données thermodynamiques sur le système Cs-O-H
- Données thermodynamiques sur le système Cs-I
- Thermodynamique du système CsI-CsOH
ETUDE CINÉTIQUE: RÉACTEUR THERMOCINÉTIQUE CHIP…
- Conception du réacteur
- Descriptif du réacteur thermocinétique
- Régime d’écoulement
Ionization cross sections of atoms were parameterized according to the ionization potential (V) in the IUPAC report[10]. The temperature was then kept constant for approximately (1 to 2) h, leading to melting of the sample. Accurate analysis of all fragment ions that contribute to the ionization process of molecules.
According to our visual observation, the uncertainty on the outflow opening portion is estimated at 0.05%.

CRITICAL ASSESSMENT OF THERMODYNAMIC DATA FOR CSOH
INTRODUCTION
This work is part of the CHIP analysis program (under the International Source Term Program), the aim of which is to analyze with a high temperature mass spectrometer (HTMS) the behavior of gaseous vapors in conditions as close as possible to those of the high temperature rupture of a primary cooling circuit of a nuclear reactor in the event of a serious accident involving a pressurized water reactor. The present study addresses the first step, namely the thermodynamic study of the complex Cs-I-O-H system chosen as the first candidate in the study of severe accidents. The present work was carried out to establish reliable thermodynamic properties for the gas phase of the Cs-O-H system, which is one of the main components transporting Cesium under excess water.
In fact, the reaction between gaseous cesium and H2O during a severe nuclear accident produces cesium hydroxide, which is one of the main components of the primary cooling lines either in the gas phase or in aerosols.
PRECEDING GURVICH ET AL. ASSESSMENT
The total ion intensities measured due to the ionization of the CsOH(g) molecule (sum of Cs+ and CsOH+ ions) were taken into account, as opposed to the dimmed intensity attributed to the single Cs2OH+ ion. Our recent determinations [1] (4 experiments) of the dimmer to monomer pressure ratio show that it varies from 0.14 to 0.6 within the above temperature range and this feature leads to a slightly different value for the dimerization enthalpy compared to Gurvich et al. Finally, Gurvic et al. the proposed values for the enthalpies of formation of the gaseous monomer and the dimmer are based on choosing the mean value of the ratio p2 / p1 and both. given that the collected values are largely scattered, Gurvich et al. the estimated mean values appear to be suggested with rather optimistic uncertainties.
Original data leading to the dissociation energy of the Cs-OH bond were reviewed by Gurvich et al. flames [5-7], HTMS/ionization processes [9-12], ab-initio calculation [13]) using third-law calculations based on their selected data (Cp°, S°T and Fef°T , given with uncertainties).
CRITICAL ASSESSMENT OF THERMODYNAMIC DATA FOR THE GAS PHASE
- CsOH(g) flames studies
- Mass spectrometric studies
The last column corresponds to the calculated third law enthalpy for the sublimation of the single monomer (CsOH(cr,l) = CsOH(g)). So the determination of Blackburn and Johnson cannot be used to determine the equilibrium dimming constant. From the corrected partial pressure of the monomer, the sublimation enthalpy according to the third law for the reaction CsOH(cr,l) = CsOH(g) was calculated to: ∆sublH standard deviation) kJ.mol-1.
The last column corresponds to the calculated third law enthalpy of sublimation of the monomer CsOH(cr,l) = CsOH(g).
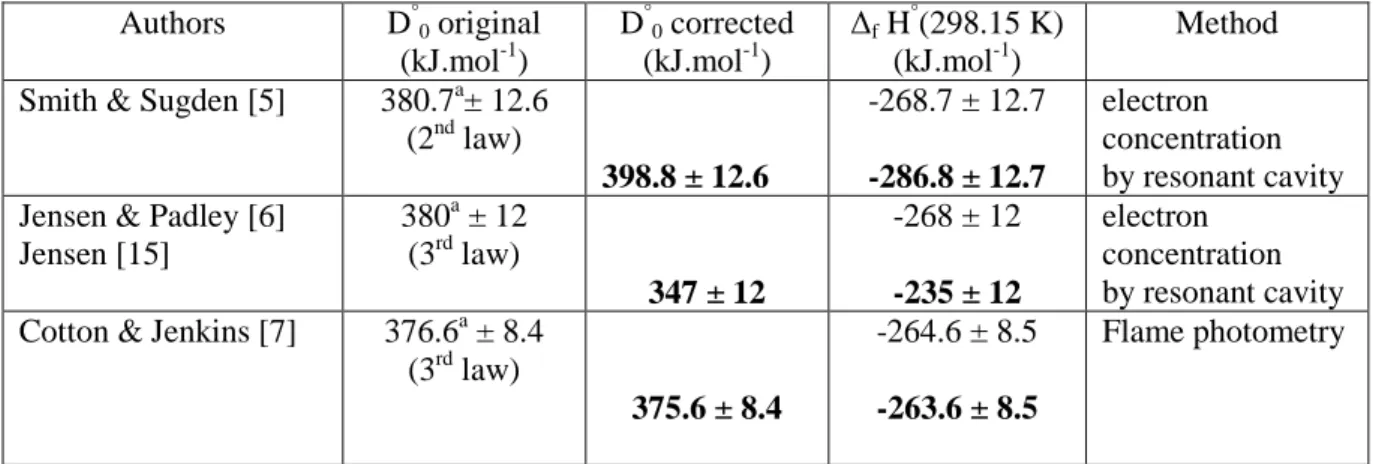
SUMMARY AND CONCLUSION
The difference in monomer pressures can be calculated using the third law relationship. Some new results could change the free energy function and consequently the dimming dissociation energy. 1] F.Z.Roki, M.N.Ohnet, C.Chatillon, and D.Jacquemain, "Thermodynamic study of the vaporization of CsOH(s,l) by high-temperature mass spectrometry," J .
Vapor pressure data are mainly evaluated to derive enthalpies of formation of the monomer CsI(g) and the quencher Cs2I2(g).

INTRODUCTION
Thermodynamic data for the Cs-I system, including solid, liquid and gaseous substances, are important for the calculation of iodine release in a severe nuclear accident from two points of view: - (i) for a final assessment of the nature of the compounds formed by iodine, and - (ii ) to scale the kinetic data that are important in the calculations of the intermediate states that occur in the primary cooling line. This study is a literature-based critical analysis of the available thermochemical data for the entire Cs-I system. Thermodynamic data for the cesium-iodine system were first analyzed by Brewer and Bracketts, then by Feber and Glushko et al.
These thermodynamic data need to be revised to propose new reliable data to be used in a consistent way for the iodine transport calculation within the framework of the CHIP1 program.
CONDENSED PHASES DATA PREREQUISITES
The value chosen by Cordfunke is in perfect agreement with the value previously recommended by Glushko et al. Measurements by Cordfunke and Prins show two different data sets: data up to about 560 K are smoothly related to the low temperature heat capacity measurements by Taylor et al. al. For the solid phase, the recent values proposed by Cordfunke and Prins are in agreement with Glushko et al.
The entropy of solid CsI has been derived from the low-temperature heat capacity measurements: by Sorai et al.

DISSOCIATION ENERGY DETERMINATIONS
The published values are largely scattered due to the various assumptions necessary in the interpretation of the results: choice of a simplified set of reactions as well as equilibrium state assumption in flame studies, assumptions about the electronic states of products or about the absence of kinetic energy of neutral fragments . In flame studies, the relatively high temperature range (≈ 2250 K) of the measurements does not guarantee the reliability of the used free energy functions as established from low temperature observations. Moreover, the presence of ions and excited states is not often taken into account either in the measurement method or in the set of considered reactions.
Following Glushko et al., these spectroscopic measurements are generally considered a first and a rough approach to the enthalpy of formation, and thermochemical determinations are needed to obtain more reliable thermodynamic functions.
TOTAL PRESSURE DETERMINATIONS
- Knudsen effusion method
- Transport/transpiration methods
- Total pressure measurements
- Discussion
The Knudsen effusion method has been used by Venugopal et al to obtain vapor pressure values over solid CsI in the temperature range 753 to 897 K assuming no damper contribution (estimated by the authors to be less than 1% at T< 863 K). Given that the proportion of the dimmer over the solid would be very low (evaporation coefficient ≈0 for example), the pressure step at the melting would be mainly due to the sudden dimmer contribution to the total pressure. This is the significance of the 11 K difference observed between the maximum temperature at the step-like (916 K) and the retained melting point (905 K) in the compilations.
Thus, according to the general development of the data in Figure III-4, Cordfunke's original solid pressure data will be selected in the temperature range 779-855 K, and this last corrected data will be retained in the temperature range 864-904 K.

CRITICAL ANALYSIS OF CSI(S,L,AND G) COMPOUNDS THERMODYNAMIC
- Proposition for a new equilibrium constant of dimmerization K dim
- Equilibrium constant for the dimmerization 2CsI(g)=Cs 2 I 2 (g)
- Third law enthalpies for vaporization reaction CsI(s,l)=CsI(g)
- Selection of the CsI(s,l) monomer enthalpy of vaporization
- Selection of the melting enthalpy and temperature
- CsI(liquid) heat capacity selection
The thermodynamic properties of the liquid depend on the thermodynamic properties of the solid by selecting the melting enthalpy CsI(l) and the heat capacity CsI(l), these two quantities being included in the calculated free energy function CsI(l) used in the third law analysis of the measured pressures above liquid. A change in the melting enthalpy of CsI(s→l) directly affects the vapor pressure, as shown in Figure III-7, while a change in the heat capacity of the liquid is a second-order effect. For this reason, we chose the average CsI(g) enthalpy of vaporization of the solid phase based on efflux studies.
After correction with the new free energy function of the fluid phase, the pressure data for each experimental method were recalculated and the results are presented in Figures III-13, III-14 and III-15.
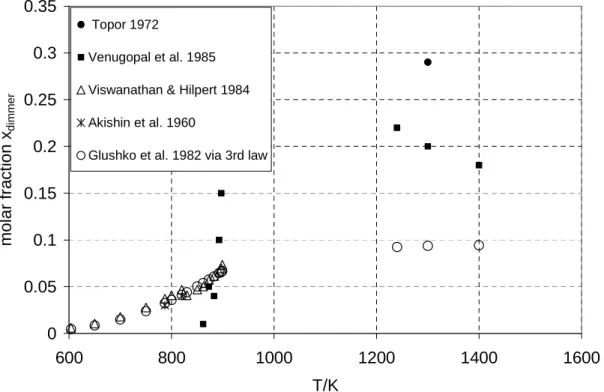
CONCLUSIONS
45] C.T.Ewing and K.Stern, "Equilibrium Evaporation Rates and Vapor Pressures of Solid and Liquid Sodium Chloride, Potassium Chloride, Potassium Bromide, Cesium Iodide, and Lithium Fluoride," J. 47] F.Z.Roki, M.N.Chatillon, C.N.Ohnet, C.T. and D.Jacquemain, "Thermodynamic study of the CsOH(s,l) vaporization by high temperature mass spectrometry," J. 56] M.Tmar and C.Chatillon, "Refinement of the vapor pressure in equilibrium over the InP and InAs compounds by mass spectrometry , J.
64] C.T.Ewing and K.Stern, "Evaporation Kinetics of Solid and Liquid Silver, Sodium Chloride, Potassium Bromide, Cesium Iodide, and Lithium Fluoride," J.

152
We attribute this anomaly to surface freezing due to high flow velocity. Calculation of monomer and dimer partial pressures combined with preliminary Rodebush-Dixon total pressure determination. Dilute fraction estimated from total pressure (boiling data) and mass transport in the 1240–1400 K temperature range.
2nd and 3rd laws did not agree due to "consistencies in thermodynamic data" (sic).
156
INTRODUCTION
In the quaternary Cs-I-O-H system, the pseudo-binary CsI-CsOH system is a section with high thermodynamic stability. In the case of gas-liquid mixtures, the reactions at the liquid surface are usually very fast, while gas-solid reactions may be "delayed" (or . "inhibited"). Further in the condensation processes, the phase diagram of the CsI-CsOH system is an important tool for understanding the condensation mechanisms and the production of liquid droplets or solid aerosols.
The complex gas phase of the Cs-I-O-H system was observed by high-temperature mass spectrometry (HTMS) by evaporation of CsI-CsOH(l) mixts.
EARLIER WORKS
- Blackburn and Johnson (1988) preceding study
- Analysis of Blackburn and Johnson Thermodynamic data
These molar fractions of the flow out - with or without taking the new molecule into account - are compared with those of the liquid X(CsOH) in figure IV-2. This stability is related to the nature of the molecular flow extremum at the congruent assembly. In figure IV-3 the total molecular flow is displayed and the congruent composition corresponds to a maximum of the molecular flow when the new molecule is taken into account.
This characteristic property is directly related to the partial pressure of the new Cs2IOH(g) molecule, which probably has its maximum value for X(CsOH) ≈ X(CsI) ≈ 0.5 (Fig. IV-1).

PRESENT MASS SPECTROMETRIC VAPORISATION DATA
- Sample preparation
- Ionization processes and gas phase composition
- Vapor pressure determinations
- Calculations assuming no new species in the gas phase
- Calculations assuming a new species in the gas phase
For CsOH (Fig. IV-7), the trends in the ionic intensity ratio (at constant temperature) are consistent with the thermodynamic evolution of the activity with composition. Figures IV-13 and IV-14 show this equation as a function of the reciprocal of the temperature of a liquid at low CsOH concentrations. Then the remaining measured intensities are attributed to the ionization of the single Cs2IOH(g) molecule.
To take into account the contribution of the CsI+ and CsOH+ fragment ions in the total ionic intensity derived from Cs2IOH(g) ionization, we calculated these contributions from figures IV-14 and IV-15, respectively.

THERMODYNAMIC FUNCTIONS OF THE CS 2 IOH(G) MOLECULE
- Structure of the molecule
- Molecular vibrations
- Other molecular parameters
- Thermodynamic functions
Remaining difference with the double-corrected values of Blackburn and Johnson (of selected Cs2O2H2(g) and monomer pressure) comes from the only retained dimer ions in their study which underestimates the actual pressure of the mixed. By analogy with the two dimmers Cs2I2(g) and Cs2O2H2(g), we said that the molecule is connected by ionic type bonds and its minimum energy as a function of the structure can be according to the Pauling's model for molecules of the family be calculated. Alkaline-halogen as NaCl, and its dimmer, etc..[16, 17]. Where E is the potential energy, r distance of separation of the two ions (anion and cation), z the ion charge (+1 or -1), e the charge of the electron, β a constant, B0 a constant so that nB0= 0.291, , r+ and r- constants characteristic of the ions and equal to their crystal radius, n a constant characteristic of the alkali halide derived by derivation of this ratio relative to r (the distance between the two ions).
The extension of the model to dimmers has previously been done by Milne and Cubicciotti and the basic relation becomes for pure dimmers.
SECOND AND THIRD LAW ANALYSIS
CONCLUSION AND PERSPECTIVES
200
NTRODUCTION
CONSTRUCTION OF THE CHIP REACTOR: PRELIMINARY CONDITIONS
- Molecule sources
- Gas flow and vapours conditions in CHIP reactor
- Choice of materials and corrosion conditions
CALCULATION OF GAS AND VAPOURS FLOW MODES IN THE CHIP
- Flow regimes
- Calculation tests for the nozzles
- Calculation of the source lines upstream pressure range
- Calculation of molecular flow between the cracking cell and the condenser
- Pressure at effusion from known gas pressure in the introduction line
FLOW PARAMETRISATION FOR SPECTROMETRIC EXPERIMENTS
THERMAL TEST OF THE CHIP REACTOR
POSITIONNING TEST OF CHIP REACTOR
PRESSURE CALIBRATION TESTS OF THE CHIP REACTOR
- Check of the mass spectrometer response for each injection line
- Calibration tests with pure components at their melting temperature
- Ar pressures at the melting temperatures
CONCLUSIONS AND PERSPECTIVES