In Run II where the total energy of the LHC has been increased, so many opportunities arise to look for phenomena beyond the SM. Due to the high mass of the graviton, the decay products of Z bosons increase greatly. The rest of the events are again passed through the FastJet to form two smaller jets, from which it selects only the events with a pair of jets with an invariant mass close to the mass of the Z boson.
Motivation
Purpose
The model 2
Kaluza-Klein theory
In the ADD scenario, the weakness of gravity compared to the other forces finds an explanation in the fact that gravity is diluted in the large volume of the extra dimensions. However, this solution simply translates the Hierarchy problem into the problem of the difference between the large size of the extra dimensions R ' 1mm and their natural value R'lP l'1033cm. The model presented in 1999 by Lisa Randall and Raman Sundrum [2] provides a new explanation of the Hierarchy Problem.
The Randall-Sundrum Model
This problem arises in quantum field theory because of divergent quadratic corrections to the mass of the Higgs field which require an extraordinary adjustment to obtain the expected mass of a few hundred GeV. The hierarchy between the four-dimensional Planck MP l'1019GeV scale and the M W TeV weak interaction scale would in reality be only apparent. The phenomenology of these models is quite distinct from that of extra large dimension scenarios; none of the current restrictions on theories with very large extra dimensions apply.
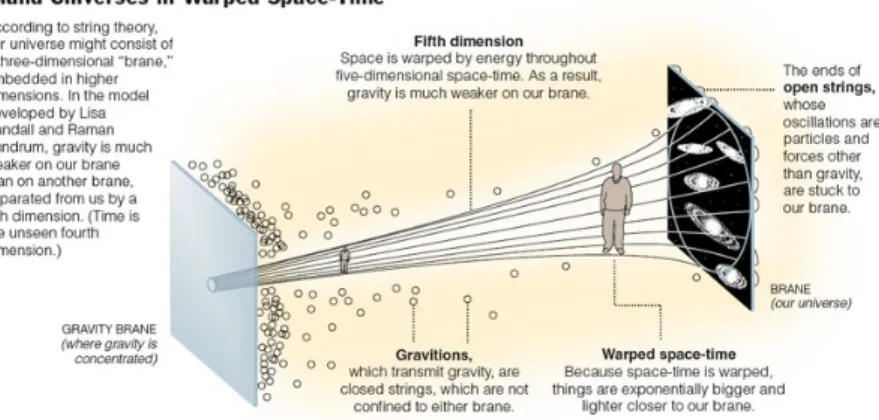
ATLAS Detector 6
The Calorimeter
Calorimeters main purpose is to measure the energy a particle loses as it passes through the detector by absorbing it. It is usually designed to completely stop or absorb most of the particles coming from a collision, forcing them to deposit all their energy into the detector. Hadronic calorimeters sample the energy of hadrons (particles containing quarks, such as protons and neutrons) as they interact with atomic nuclei.
Muon Spectrometer
Magnet System
Event Generator 12
The Simulation of Hard Processes
The phase space is a multidimensional hypercube that encloses the entire degree of freedom. The dominant use of the first method is to create histograms that are consistent with the physical distribution. A histogram of some relevant distribution (e.g. the d-quark transverse momentum) is populated with the weighted events of candidate events.
For this process, the maximum occurs when one of the final state quarks is collinear with one of the initial state quarks, so it is easy to calculate dσM AX by inserting these states (cosϑ =±1) into eq.(5) add. The accepted events have the frequency and distribution predicted by eq.(5) and represent the physical expectation for the imaginary u¯u collision experiment. However, the process in eq.5 is not physical, because the kinematics of the process can be trivial (eg Z0 has zero momentum) and also because we cannot have quark beams and detect isolated quarks.
There is no description of the radiation of any extra particles, since the number of particles is fixed, both in initial and final state. So in the case of the trivial kinematics, the extra radiation on top of the hard subprocess has to do with the consideration of higher-order corrections in perturbation theory, while the case of isolated quarks is a way to find a way to to describe the connection from the quark to a state we can observe in nature (hadronization). Regarding the estimation of the dominant effects due to emissions at all orders, there are two different approaches.
The second procedure forms the basis of the Parton shower technique and is, by construction, the core of event generators.
Higher order correction
- Hard Subprocess
- Parton Showers
- Hadronization
- Underlying Events
Simulating the hard process is relatively difficult because Parton Distribution Functions (PDFs) describe partons entering the process, and the lowest-order perturbation theory gives a probabilistic distribution of the outgoing partons. Convolution with the differential cross-section of the sub-process and integration over phase space yields the relevant production cross-section. Any of the incoming or outgoing objects of the hard subprocess that carry QCD color are eligible for parton showing in the next phase of the simulation.
After the particle shower ends, partons remain with virtualities on the order of the cut-off scale. Each of these corresponds to one of two parameterization models: the string model and the cluster model. As the partons move further apart, the potential energy of the string increases until it is large enough to produce aqq¯ pair.
The occurrence of a pair breaks the string in two according to a parameterized fragmentation function. This procedure reproduces the expected steeply decreasing distribution of mass clusters, with the primary dependence only on the threshold value of the parton shower. The presence of these particles, although not directly related to the hard collision, can affect the interpretation of the data.
In a proton-proton collision, the resulting event contains particles originating from the two outgoing partons (plus initial and final-state radiation) and particles originating from the breakup of the initial hadrons (i.e., jet remnants).
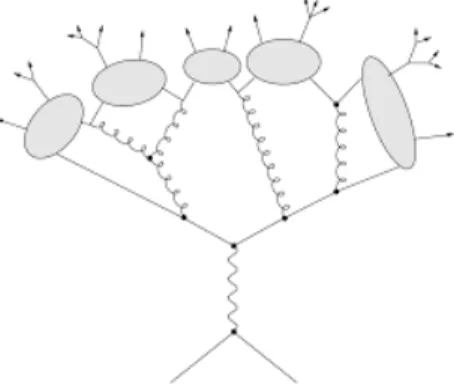
MadGpaph - MadEvent 21
Overview
As a result, both MadGraph5 and the code it produces run significantly faster than previous versions. More importantly, however, MadGraph5 includes the implementation of a new color representations (color sextets and eijk), implementation of multifermion vertices, and addition of new output formats, including output in C++ and Python.
Pythia 23
Overview
Jet algorithm - FastJet 25
Jet reconstruction
The sequential recombination beam algorithms, (such as anti-kt algorithm), which set a distance measure between particles, and repeatedly recombine the nearest pair of particles until some stop measure is reached. Cone beam algorithms are based on identifying the energy flow in cones in pseudorapidityη =−lntanθ2 and azimuth φ, merging and matching the cones to obtain the final beams. A disadvantage of the cone algorithms is that different experiments used different variables and it is often difficult to know exactly which beam finder to use in theoretical comparisons.
On the other hand, the sequential recombination beam algorithms are based on sequential pairwise recombination of particles and using the same variables in different experiments.
The anti-kt algorithm
FastJet
Furthermore, it provides the necessary tools to assess the density of noise pollution in an event and to extract the appropriate amount of noise from each jet. The interface here shares a number of characteristics with the underlying tools, some of which also serve to remove noise pollution. Both the substructure and pile removal also make use of a selector frame to specify and combine simple cuts.
Purpose
Background processes
Steps for creating the processes
In the first step, where the processes are generated using Madgraph, we set the parameters shown in the tables. This file is scanned for all events and all values and parameters are stored in histograms. In the second step, the PYTHIA6.8 program is used to perform the parton shower and hadronization.
Then, after showering, an STDHEP text file is produced (.hep format) containing all the particles in each event. The shower procedure produces hundreds of 'final state' particles, each of which is represented by a Lorentz 4 vector and is representative of the particles entering the ATLAS detector. Because there are so many particles in this final state, these files have been formatted in accordance with the Les Houches Agreement so that they meet the standards of the particle physics community. There is well-developed software that can read them and put them in the computer. conditions of a root format file.
Fastjet uses as input only the vector with four moments of the particles in each event. Fastjet is asked to perform the antique algorithm for all jet events with inclusive pT >25GeV. For each beam, it gives pT, the energy, mass, and velocity of the beam.
Our goal is to reduce the number of events arising from background processes, to achieve that we:
Choice of the appropriate variables
- Jet Radius
- Other variables approprite for selection
Our goal is to choose the most efficient combination of the radius Rj1 and Rj2, in order to achieve the maximization of the number of gravitons created. Out of all these cases, it is worth seeing in detail the two most effective choices, namely the 1) and the 4). The following table shows the sigma of the mass distribution in all the previous cases with Rj1=1 and Rj1=0.6 and Rj2=0.4.
According to the previous plots and table, it is clear that we have better distribution in the case of the combination Rj1=0.6 and Rj2=0.4. Nevertheless, this is not our goal, so to be completely clear which combination is the most efficient, we need to calculate the number of gravitons "reconstructed" in each of these cases. After selecting the combination of beam radius, we can continue our analysis. The following histograms show the invariant mass distribution of the one FatJet and the two Klein jets and the mass of the graviton in these two.
Our goal is to find the value that can give us both good discrimination of the signal process and good rejection of the background process close to the Graviton region. The following figures show the efficiency of the signal and the rejection of the background as a function of the cuts we have used. To conclude, after seeing all the previous plots, we came to this "decision", we use Rj1=1 and Rj2=0.4 jet radius to choose the maximum number of gravitons that we can, and we apply the jet mass cut equal to with 25 GeV and that because this cut gives us the maximum efficiency for our signal process and at the same time the maximum rejection for the background processes.
After we complete the analysis for the jets, we need to look more closely at muons, which will provide the invariant mass of the second Z boson. Mass of the Graviton 900≤mllJ ≤1100 and 900 ≤mlljj ≤1100 After applying these reductions we can see in the following table the proportion of generated events remaining in each channel. Now we have finished studying the cuts and can see the results in the following histograms.
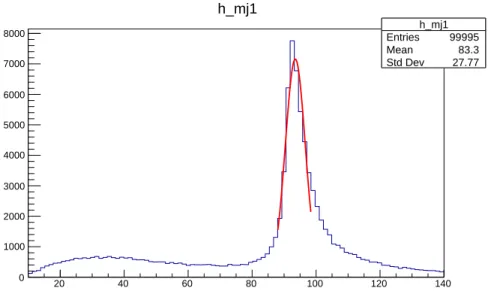
Conclusion 56
1] https://plus.maths.org/content/kaluza-klein-and-their-story-fifth-dimension [2] Maxime Gabella, The Randall-Sundrum Model. 4] The ATLAS detector http://atlas.cern/discover/detector [5] ATLAS-eksperiment https://en.wikipedia.org/wiki/ATLAS. 8] Fabio Maltoni and Tim Stelzer, MadEvent: Outomational Event Generation with MadGraph https://arxiv.org/pdf/hep-ph/0208156v1.pdf [9] Johan Alwall, Michel Herquet, Fabio Maltoni, Olivier.