I hereby declare that I have not submitted this Master's thesis in any form (for review to a reviewer), neither in Austria nor abroad. The aim of this study was to investigate whether the size of a bacterial aggregate (biofilm) affects the ability of polymorphonuclear leukocytes (PMNs) to eradicate them. PMNs are part of the innate immune system and are the host's first line of defense against numerous infectious pathogens.
The most important clinical characteristics of biofilms are an extreme resistance to antibiotics and a great ability to evade the immune system. It is not yet understood why PMNs of the human immune system fail to eliminate some biofilms when they first form. The results showed that the size of the PMN itself is the limiting size for phagocytosis.
I NTRODUCTION
- P LANKTONIC VS . AGGREGATION
- T HE B IOFILM IN CHRONIC INFECTIONS
- B ACTERIA
- Escherichia coli
- P SEUDOMONAS AERUGINOSA
- S TAPHYLOCOCCUS EPIDERMIDIS AND S TAPHYLOCOCCUS AUREUS
- K ILLING MECHANISM OF THE DIFFERENT BACTERIA
- P SEUDOMONAS AERUGINOSA – VIRULENCE FACTORS
- S TAPHYLOCOCCUS AUREUS – VIRULENCE FACTORS
- E SCHERICHIA COLI – VIRULENCE FACTORS
- S TAPHYLOCOCCUS EPIDERMIDIS – VIRULENCE FACTORS
- PMN S
- H OW PMN S RECOGNIZE AGGREGATES / BIOFILMS ?
- P HAGOCYTOSIS
- H OW PMN S KILL MICROBES
- PMN S IN CHRONIC BACTERIAL INFECTIONS
The bacteria are immobilized in the aggregates, where they bind and bind together (see Figure 2) (Alhede et al. 2011). The clinical presentation of EAEC infection was mostly associated with acute watery diarrhea (Huang et al. 2006). The exact physiological function and mechanics of their production are still unclear (Chrzanowski et al. 2012).
Both the rhlAB operon and the rhlC gene are controlled by the rhl quorum sensing (QS) and Pseudomonas quinolone signal (PQS) systems (Rahim et al. 2001). Consequently, no C3b is produced and opsonization and phagocytosis of bacteria are significantly reduced (Higgins et al. 2006). An additional virulence factor of strain EAEC 042 is a locus encoding a polysaccharide capsule (Chaudhuri et al. 2010).
In humans, approximately hundreds of billions of PMN enter and exit the circulating blood each day (Amulic et al. 2012). Furthermore, wounds are susceptible to infection due to impaired host immune response (Wolcott et al. 2008).
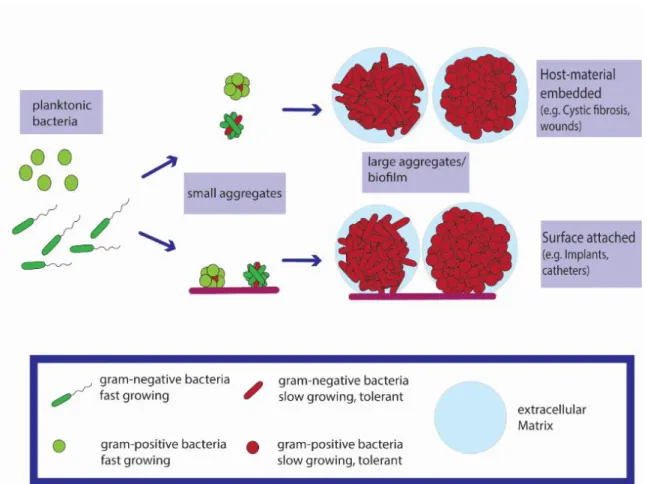
M ATERIAL AND M ETHODS
- B ACTERIAL STRAINS
- G ROWTH CONDITIONS
- M ATERIALS
- I SOLATION OF PMN S
- P REPARATION OF PMN S AND BACTERIA FOR THE MICROTITER WELL
- M ICROSCOPIC EVALUATION
- L IVE / DEAD EVALUATION
- S IZE MEASUREMENT
- S TATISTICAL ANALYSIS
The bacteria were grown in 50 ml Erlenmeyer flasks with 10 ml LB/TSB for 20 h shaking at 180 rpm at 37°C. The bacteria and PMNs were stained with Syto 9 (Molecular Probes, Thermo Fisher Scientific), a green fluorescent nucleic acid stain to label the DNA. As a dead indicator, the bacteria and PMNs were stained with Propidium Iodide (PI) (Molecular Probes, Thermo Fisher Scientific).
The blood was centrifuged at 500 g for 25 min in a swing-out rotor (RT) without soft stop or soft start, just before the centrifugation was completed (1 min.), G was increased to 700 g and another 10 min. Bacteria were grown for 20 h in shaker tubes (180 rpm) and left for 10 min on the table before 1 ml from the top of the tube was transferred to a 1.5 ml Eppendorf tube. 10 min before use, Syto9, a green fluorescent dye for live cells and PI, were added to monitor cell death.
Immediately afterwards, the microtiter plate was placed in the microscope and observed for up to 8 hours. Bacteria were grown in shake flasks (180 rpm) for 20 h and left on the table for 10 min before transferring 1 ml from the bottom of the flask to a 1.5 ml Eppendorf tube. Immediately afterwards, the microtiter plate was placed in the microscope and different positions/areas with aggregates of different sizes were defined.
To visualize the PMN's membrane and the uptake of the bacteria, we used a combination of fluorescence and light microscopy. The bacteria and PMNs were stained with Syto9 (green fluorescent nucleic acid stain, Molecular Probes, USA) for the live cells and Propidium iodide (Molecular Probes, Thermo Fisher Scientific) for the dead cells. For microscopic evaluation, 300 µl of the stained culture (OD=0.1 450 nm) was applied to 96-well microtiter plate, uncoated and sterile (Ibidi, Germany) and 100 µl of PMNs were added to give a final concentration of 1 ×106 PMNs/ml.
Aggregate size measurement (maximum range) was determined using the measurement tool in the Zeiss Zen 2010 microscope image analysis software (version 6.0; Zeiss, Germany).
R ESULTS
P. AERUGINOSA AGGREGATES T UBE VS . F LASK - O XYGEN
To determine the best way to obtain aggregates or planktonic bacteria for each strain, we cultured the bacteria in test tubes or Erlenmeyer flasks with identical facilities. In Erlenmeyer flasks, the bacteria were constantly supplied with oxygen and could easily form aggregates. When cultured in test tubes where oxygen is only present at the top, the phenotype observed was planktonic bacteria at the top and aggregated bacteria at the bottom of the test tubes.
While the aggregates from the flask were very compact and surrounded by dead bacteria, the aggregates from the tube consisted mostly of live bacteria (green) and had a fluffy phenotype (see Figure 6/B). In contrast to the aggregates from the flask, which were surrounded by dead bacteria, the PMNs could neither recognize nor, consequently, phagocytose them.
PMN S AND DIFFERENT SIZES OF BACTERIAL AGGREGATES : LIVE OBSERVATION
Rhamnolipids have been found to be agents that cause PMN necrosis (Jensen et al. 2007). The mutant strain we used was also virulent, so the mutant kills PMNs from within. Experiments were started by first adding bacterial aggregates, determining the positions/areas of different aggregate sizes (see Figure 7) and finally adding PMNs.
A migration of the PMNs towards the selected aggregates was seen, followed by a disruption and uptake of the aggregates. After 150 minutes the PMNs had no success, they died and the aggregate is still there. As seen in Figures 11 and 15, sizes of various aggregates are plotted and if the PMN(s) died or lived after engulfing the aggregate (see Figure 11).
When the aggregate was larger than 5.00 µm, the PMNs began to die after phagocytosis (see Figure 9). Interestingly, if the aggregate was larger than 15.00 µm, the PMNs failed, dying during phagocytosis (see Figure 10). When larger aggregates were used, a migration of more PMNs to those aggregates was observed within 20–60 minutes, followed by disruption and uptake of the aggregate.
But this is hard to say, because PMNs are moving, and so they often move from their fixed position making it impossible for us to follow their outcome. In this study we focused on aggregates up to 45 μm, but during our experiments we could also observe that if there is a real biofilm, then the PMNs start to give up, they start to phagocytose the biofilm, but after 18 hours the rest of the living. PMNs just lie there around the biofilm. If the bacterial aggregates are not as virulent (P. aeruginosa rhlA) using a mutant that has lost a virulence factor that is important for PMN necrosis, then PMNs can phagocytose larger aggregates.
After 140 min, the PMNs had completely phagocytosed the aggregate and remained alive (a video of these events is supplemented as S2).
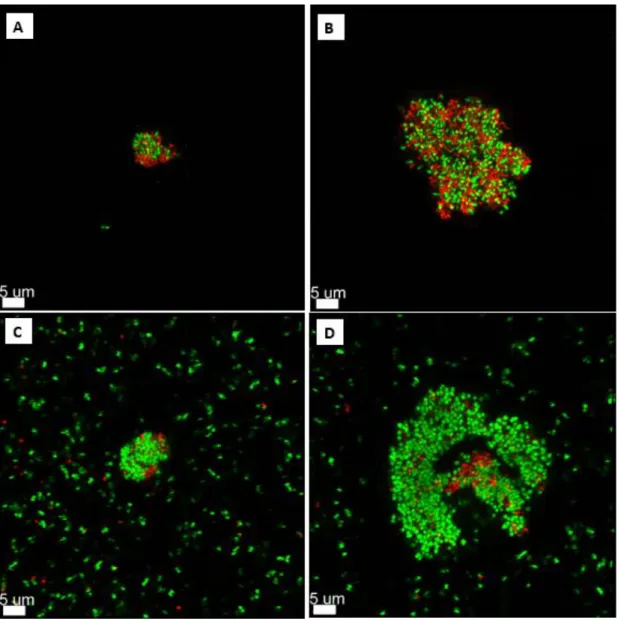
D ISCUSSION
Moreover, they raised the possibility that EAEC-induced PMN infiltration may promote the pathogenesis of EAEC in the host (Boll et al. 2012). EAEC infections are often persistent and significantly inflammatory (Steiner et al. 1998), but little is known about virulence factors that can kill PMNs. 2008) have shown that macrophages can most efficiently recognize particle diameters of 2-3 µm and exhibited maximal phagocytosis and adhesion (Champion et al. 2008).
This is an important observation because the size of 1–4 µm represents the usual size of airborne and waterborne bacterial pathogens (Kowalski et al.; The majority of research focuses on macrophages, but much less is known about the size limitation for PMN phagocytosis.. When studying whether there is a size limitation for phagocytosis, activation of host defense cells was seen with massive infiltration of neutrophils onto the aggregates/biofilm, followed by uptake of the aggregates.
The size limitation for phagocytosis depends on the bacterial species, the age of the aggregates and the virulence of the phagocytosed bacteria. 2009) have shown that clearance depends on the age of the biofilm. Furthermore, rhamnolipids were found to be the agents that cause necrosis of the PMNs (Jensen et al. 2007). But even if the bacteria are still virulent, we have shown that a single PMN can easily phagocytose aggregates of approx. 10 µm and stay alive.
PMNs were able to phagocytose the aggregates, but they died at this time and thus had no success, suggesting that the aggregate contains too many bacteria secreting diverse and apparently too many virulent factors that kill the PMNs. We show that one PMN can phagocytose aggregates approximately 14.74 µm in size, and multiple PMNs can phagocytose large aggregates/biofilm without dying. These data suggest that there is a difference in the size limit for phagocytosis that may depend on the bacterial species and the virulence of the pathogen.
Furthermore, our data suggest that the size of the PMN itself (PMNs have an average diameter of 12–15 µm) is the limiting size for phagocytosis.
C ONCLUSION
But a lot more research is needed, and we need even more research with different bacterial species.
R EFERENCE LIST
Pseudomonas aeruginosa biofilms exposed to Imipenem exhibit changes in global gene expression and lactamase and alginate production. Borregaard, Niels (2010): Neutrophils, from marrow to microbes. 1995): Pseudomonas aeruginosa biofilms: the role of the exopolysaccharide alginate. Structural studies on the O-polysaccharide core and repeating unit of Pseudomonas aeruginosa immunotype 1 lipopolysaccharide.
In: Wound repair and regeneration: official publication of the Wound Healing Society [and] European Tissue Repair Society 19 (3), P. 2001): Prevalence of type III secretion genes in clinical and environmental isolates of Pseudomonas aeruginosa. Rapid necrotic killing of polymorphonuclear leukocytes is caused by quorum-sensing-controlled production of rhamnolipid by Pseudomonas aeruginosa. Pseudomonas aeruginosa Cell-to-cell signaling is required for virulence in a model of acute lung infection.
The human pathogen Pseudomonas aeruginosa uses conserved virulence pathways to infect the social amoeba Dictyostelium discoideum. Iglewski, Barbara H.; Hamood, Abdul N. Contribution of quorum sensing to the virulence of Pseudomonas aeruginosa in burn wound infections. Structural characterization of the outer core and the O-chain linkage region of lipopolysaccharide from Pseudomonas aeruginosa serotype O5.
1996): Contribution of specific Pseudomonas aeruginosa virulence factors to pathogenesis of pneumonia in a neonatal mouse model of infection. In: The International Journal of Artificial Organs 28 (11), S. 1998): Cell-to-cell signaling and Pseudomonas aeruginosa infections. of Gennip, Maria; CHRISTENSEN, LOUISE DAHL; Alhede, Morten;. Inactivation of the rhlA gene in Pseudomonas aeruginosa prevents rhamnolipid production, which disables protection against polymorphonuclear leukocytes.
Alginate is not a significant part of the extracellular polysaccharide matrix of PA14 and PAO1 Pseudomonas aeruginosa biofilms.
L IST OF FIGURES
S UPPLEMENTARY MATERIAL