Subsolidus- und Schmelzexperimente wurden bei 2,0–3,7 GPa und 750–1300 °C in KCaMAS-HC (Kapitel 4) durchgeführt, um die Stabilitätsfelder von K-Glimmer zu bestimmen und die in der Flüssigkeit herrschenden Schmelzbedingungen zu ermitteln. Das anfängliche Schmelzen wird durch die Reaktionen Phengit+Zoisit+Quarz=Klinopyroxen+Kyanit-Schmelze (>2,5 GPa) und Biotit+Zoisit+Quarz(±Amphibol) - Schmelze+Klinopyroxen+Kyanit (<2,5 GPa) verursacht.
General introduction
Thomsen TB and Schmidt MW (in review) Biotite to phengite transformation and mica-dominated melting in carbonate-saturated pelites at high pressures: implications for. Thomsen TB and Schmidt MW (2004) Biotitophengitic transition and mica-dominated melting in carbonate-saturated model systems.
Micas: crystal chemistry and petrological aspects
General formula and classification
The coordination of the octahedron is completed by the A anions (the OH ions). The octahedral sheet can be composed in two directions: either predominantly of divalent cations such as Mg21 and Fe2', in which case the three octahedral sites (Ml, M2, M3, are filled in an eight shape. Fig. 2.4. Main variations and exchangers for dioctahedral and trioctahedral potassium micas, with tetrahedral occupancy (M) and planes of Si,Al and (Mg,Fc2+)atomspfu (based on 11octahedral and ¬octahedral,vlis.
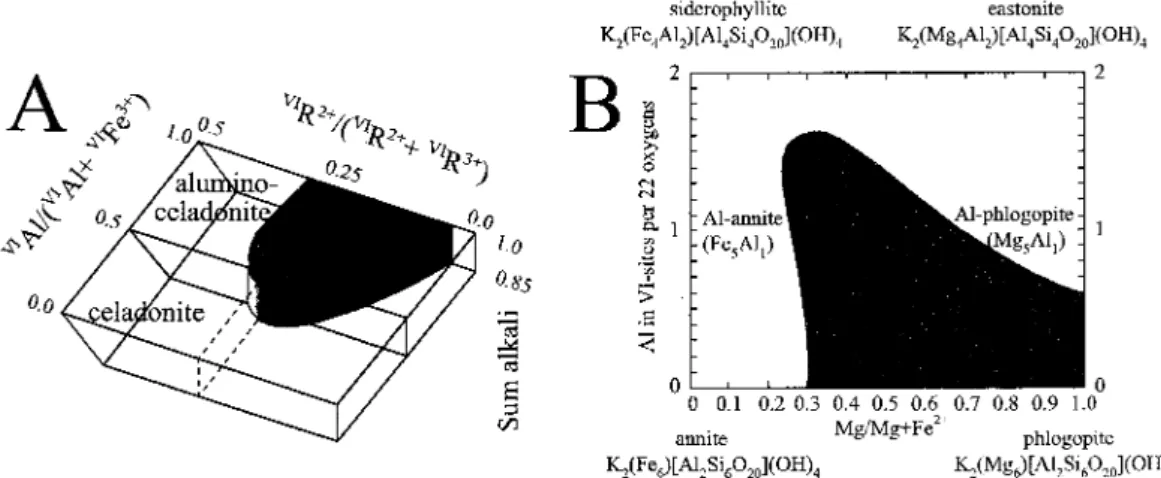
Experimental and Analytical methods
B). Capsule welding and preparation were carried out as for the piston cylinder experiments
Bulk starting materials
2. (resulting in more oxidizing conditions than boron nitride assemblies) and Fc conditioning of capsules (Fe loss of -10% (relative) in non-preconditioned capsules compared to <3% in Fc preconditioned capsules). The use of boron nitride powder (in combination with MgOliners and MgOoctahedra) in the multi-anvil experiments, compared to MgO alone in the piston-cylinder experiments, could also be considered.
Approach to equilibrium
After the capsules are deflated, the capsules are assembled lengthwise, and the capsules are dissolved and polished in the direction of the capsule to form the center of the capsule, impregnated and then finely chopped. Most experiments were polished by an automated method using acidic silica colloid.
Analytical techniques
- Electron Microprobe analyses (EMPA)
- Scanning Electron Microscope (SEM)
- Starting material
- Analytical techniques
For MSCARLO, the standard deviation(la) of individual oxide concentrations obtained from the microprobe analyzes of each phase was used to calculate the error of the phase ratios that best fit the proportions calculated by the MSROCK regression procedure. The number of replicates for each experimental load was between 10,000 and 10,000. Phengite controls fluid-saturated melting at >2.6GPa, while biotite dominates at <2.4 GPa (and 2 GPa also amphibole). The solidus is located at 850-950°C,.
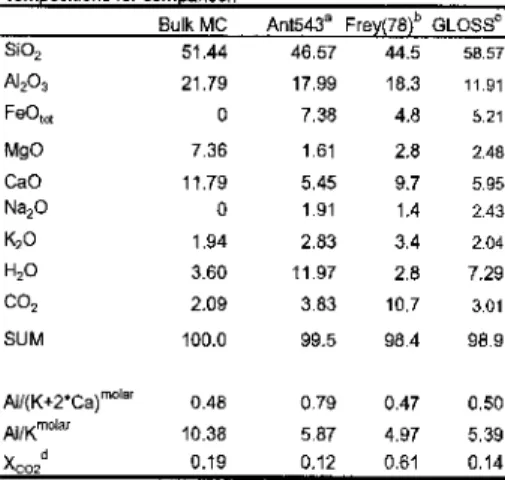
Experimental results
- Crystallization and phase compositions
Zoisite is stable to a few 10 °C above the solidus, i.e. to ~ 900 °Cat2.5 GPa and to 970 °Cat3.5GPa, in this pressure range zoisite is the last hydroxylated phase to disappear through melting. Clinopyroxene is present in almost all experiments of this study, except in the lower pressure and temperature region (<875 °Cat2.0 GPato<750 °Cat2.5 GPa), where amphibole replaces clinopyroxene; amphibole is restricted to this part of the P-Tgrid. Garnet emerges between 950–1050 °C and is stable to the liquid state at 1300 °C (2.5 GPa). The experiments result in three distinct regions: Aphengite and biotite testability field separated by an arrowmica transition zone between 2.4 and 2.6 GPa, at higher temperatures mica no longer exists and silicate melts are produced, coexisting with clinopyroxene, kyanite and.
In this field, phengite is the only potassic phase present and coexists with clinopyroxene, zoisite, dolomite, kyanite, coesite/quartzandane in excess of H2OC02 (Fig. 4.3A). Below the mica transition zone, phengite is absent and several stability fields of the presence of biotite can be determined with slightly different coexisting phase associations (Fig. 4.2, 4.3C+E, Tab. 4.2). The GPa increases slightly up to 0.2 Mg pfu, implying a smaller octahedral void than in the ideal pseudobinary phengite solid solution (Figure 4.4A). This feature is supported by the lower content of Almand Sipfu than in ideal phengites (Fig. 4.4B) and can be attributed to the exchange along the dioctahedral-trioctahedral substitution of mica ( Mg^A^Q, ) between muscovite and phlogopite. In the KCaMAS-HC system, ideal biotite is formed due to Mg-tschermak substitution (Mg, Si, Al2) in the phlogopite-castonite solid solution, i.e. KMgJAISiJO,„(OH)2-KMg2Al[Al2Si2l O10(OH)2. The total cations for the analyzed biotites range between 7.35 and 7.66 apfu with octahedral spaces of 2.55 to 2.82 pfu and interlayer voids of 0.11 to 0.28 pfu, indicating non-ideal biotites with deficient interlayers. acation and octahedral voids from 0.18 to 0.45 pfu (Figure 4.4A). With increasing pressure and decreasing temperature of potassium.
C(2.5GPa). Above the solidus, silica content decreases with temperature giving an increase in alumina on the tetrahedral site from 0.01 Alpfuat at lower temperatures (850°C) to 0.30 Alpfuat 1200 °C. Supported by the decrease in Mg with an increase in an increase in the temperature , Si, Al2) inclinopyroxenes with temperature (Fig .4.5A). Calculated M2 site vacancies amount to 0.03-0.08pfu corresponding to 6.3 -16.2 mol% Ca-escolaite, which increases with pressure at constant temperature. Experimentally produced clinopyroxenes with 4-9 mol%. The same relationship between M1, excess Al pfu and total cation deficiency is observed in this study. Overall, a comparison of Ca-eskolite component in clinopyroxenes from this study with the experimental results of Hermann (2002), Schmidtetal. (2004), and natural water presses of 5% of the soil areas (5% of the soil) Pa and 1100°C, Schultzeetal ... not at the highest.
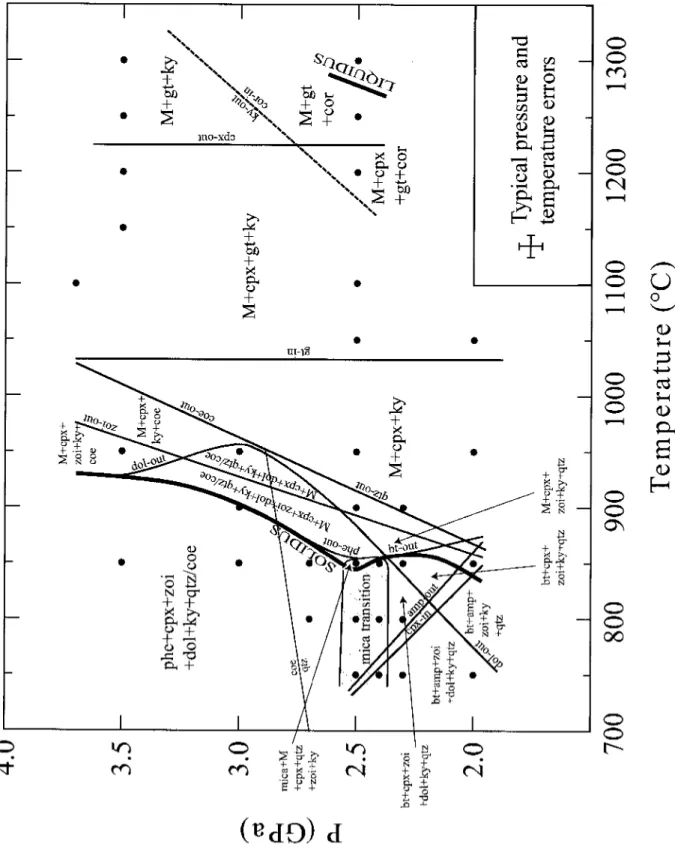
B). The structure of zoisite was confirmed (with respect to clinozoisite) by micro-Raman
- The transformation reaction of biotite to phengite
- The liquidus surface
Mineral-melt fractions in the biotite-phengite reaction in a nearly isothermal section below the solidus at 750–850 °C and along isobaric sections at 2.5 and 3.5 GPaarc. This suggests a K20-conservative biotite-phengite reaction buffered by zoisite, quartz/coesite, clinopyroxene and kyanite. The experiments allow the quantification of the decomposition reaction from the analyzed phase compositions only within the stability field of phengite (at 2.7 GPa) and biotite (at 2.3 GPa) at 800 and 850 °C:. reaction coefficients sarcmolar, melt normalized to 8 oxygens). The reaction necessarily preserves K20 since no other K-bearing phase is observed, but somewhat surprisingly it is reaction (1). The amount of fluid produced from zoisite (~0.5 wt% H.0 in our experiments) would either result in a decrease in Xco in the fluid or some concomitant decarbonation of the dolomite, but the latter is within error in our mass balance.
Both the amphiboletoclinopyroxene and biotite ctophengite transformation reactions show that zoisite is most abundant in the low-temperature-low-pressure part of the P-T grid (from 40 wt% at 750°C, 2.0–2.3 GPa down to 9 wt% at 850°C , 2.5-3, in a first area). reduced by -10% relative to the amphiboletoclinopyroxene reaction, so. Toward higher temperatures, melt fractions increase only moderately until significant incoherent melting of clinopyroxene+cyanite begins (Fig. 4.7B+C), forming garnet through the reaction (at 2.0-2.5GPa). The melting ratio defines the liquid surface of the KCaMAS-HC system, projected from quartz/coesite, kyanite and liquid, thus leaving the main components MgO,CaO and K?0 (Fig.4.6C).
In our bulk composition, phengite melts first and fluids then develop along the peritectic line zoisite=clinopyroxcne+fluid. Both the peritectic PM and the peritectic line at higher temperature shift toward K20 with pressure, resulting in an unclear difference in the mclt composition between the experiments at 3.25 and G.0-3.252. It should be noted that both clinopyroxene and garnet vary in their composition along the MgO–CaO edge of the triangle, clinopyroxene becoming more aluminous through schennaks exchange mainly with temperature (Fig. 5A) and garnet becoming more magnesian with pressure.
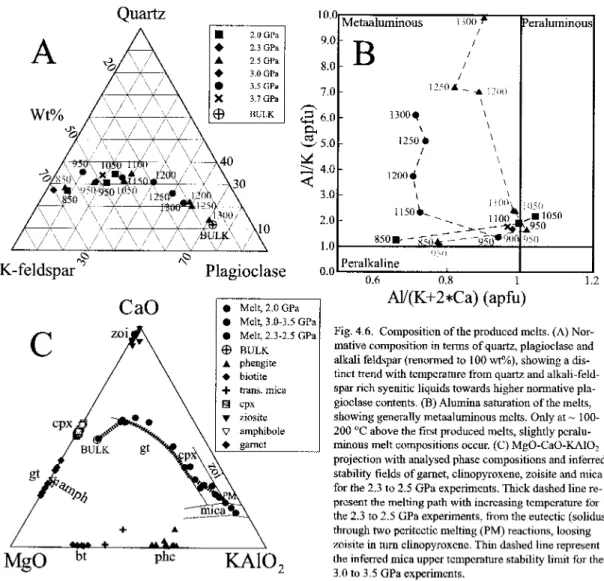
Comparison of the P-T grid to previous high pressure expérimentai studies
- Experimental procedure
- Analytical methods
Experiments at KCMASAt2.0-4.5GPa,680-1050 °C with 2-5 wt%H30(Hermann produced biotite and phengite coexisting from 2.5 to 3.9 GPaat780 °C, This pressure range is narrow towards higher temperatures high. Fig. 2).The stability range of aphengite above 2.5 GPa is consistent with our results. band for the H20-saturatcdtonalite melting experiments of Schmidt(1993) (Fig.4.8 ad.8)at-1.3GPa(and 650 . °C) coexisting with amphibole+plagioclase+epidote+quartz and in the H20 experiments (20005 unsaturated) Fig.4.8 ad.9) at 1.7-1.9 GPa (and 800-900°C) coexisting with Kfsp+cpx+garakt+quartz+melt+kyanite.
Hermann&Green (2001) and Hermann (2002) than in the study (Fig. 4.8, ad. 2). Phengite melts at similar temperatures (at 2.5-3.5 GPa), but the upper limit of the thermal stability of biotiteat2.0-2.5 GPa is about 50 °Chigherin Hermann's experiments, which is caused by changes in the bulk composition. Fortonalité, melting above 2.5 GPa is controlled by phengite (±zoisite) (Schmidt1993, Patino Douce2005). In H20 unsaturated tonality, phengite falls to -150 °The highest temperatures, reflecting a lower mass aH0 than the bulk bulk composition, where the bulk composition is 2000D. point-dominated solids at 600-700 °C ( Schmidt, 1993),duetoahigheraH„.Biotite generally breaks lower pressures, in H20-undcrsatu-.
Experiments at 1.5 to 3.5 GPa and 700–1000 °C on a carbonate-saturated eclogite (alteredoceanic basalt) with 10 wt% calcite (Yaxley & Green, 1994) produced just above the 700–750° strength C, silicate melts and C02-enriched fluids. Ompha citcisomnipresent, while garnet is restricted to <1.5 GPa and 850-1000 °C and damfibole to below 2.5 GPa and 950 °C. The latter is located at 900 °C, 2.4 GPa and 1070 °C, 5.0 GPa. Phcngite+quartz/coesite controls the initial silicate melting and melt productivity by the reaction phcngite+quartz/coesite+clinopyroxcnc+calcite=silicatemelt+kyanitc+garnet, leaving most of the Fe-Mg-calcicine residue e .
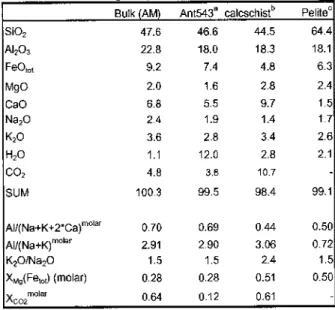
Experimental results
- Approach to equilibrium
- Phase stability and phase relations
Experiments just above diesolidus have rounded vesicles in the silicate glass (Fig. 5.2B-T), indicating the presence of some fluids at supersolidus conditions. Fe-Mg calcitsis stable to <. 1100 °Cat2.5 GPa entot1150 °Cat3.5 GPa(Fig.5.2D+G), which coexists with silicate melt above the solidus. At 5.0GPa, calcite does not occur above the solidus. Coexisting silicate and carbonatite. At the latter pressure, garnet erupts at 1100 °C before clinopyroxene and kyanite (Fig. 5.2H), while all three of them erupt between 1200 and 1250 °C at 3.5 GPa.
1150 °C (C) a single silicate melt appears. (D) An initial 3.7 GPa silicate melt coexists with rounded Fe-Mg-calcite, the latter being replaced by carbonatite melting at 1100 °C. G) Initial melting at 2.5 GPa with liquid possibly present (H) Rounded bubble in calcium-free melt stability field + gt + ky/cor. (1) Ca-rich dissolution. Clinopyroxene/silicate Na melt partitioning along the solidus increases from 2.5 to 3.7 GPa with D^px/meit-3 j ^07 3^respectively; however, it is slightly lower in the near-solid carbonatite experiment at 5.0 GPa with tj cpi/nieit=3 ^ j^&silicate melts coexist with clinopyroxenes with jadeite contents ranging from Td38 (2.5 GPa) to Jd6g61 (3.7 and 5 .0 GPa) nearby. The carbonatite melt forms <20hr, rounded blobs with wrinkled textures (Fig. 5.2B+E+F), similar to the carbonatites produced by Kjarsgaard (1998), Lee&Wyllie Kjarsgaard& Peterson (1991) and Wyllie& Turtle (1960). The wrinkled texture probably indicates Kjarsgaard & Peterson( 1991) and Wyllie&.
Below the solidus, Fe-Mg chalates form 5-25Lim, polyhedral grains. Above the solidus, the calcites become rounded (Fig. 5.2D) dumbbell-shaped, similar to the superolidus calcites of Kjarsgaard (1998), Lee &Wyllie andWyllie-Tuttle and(Ic96&Wyllie). calcites (Tab.5.6, Fig.5.6A) have molar Mg# of 0.48-0.74, which increases with temperature, and do. Terapic generation of large amounts of silicate melt (30-35 wt%) within <50 °C is controlled by the melting of phengite and quartz/coesite (Fig. 5.3).
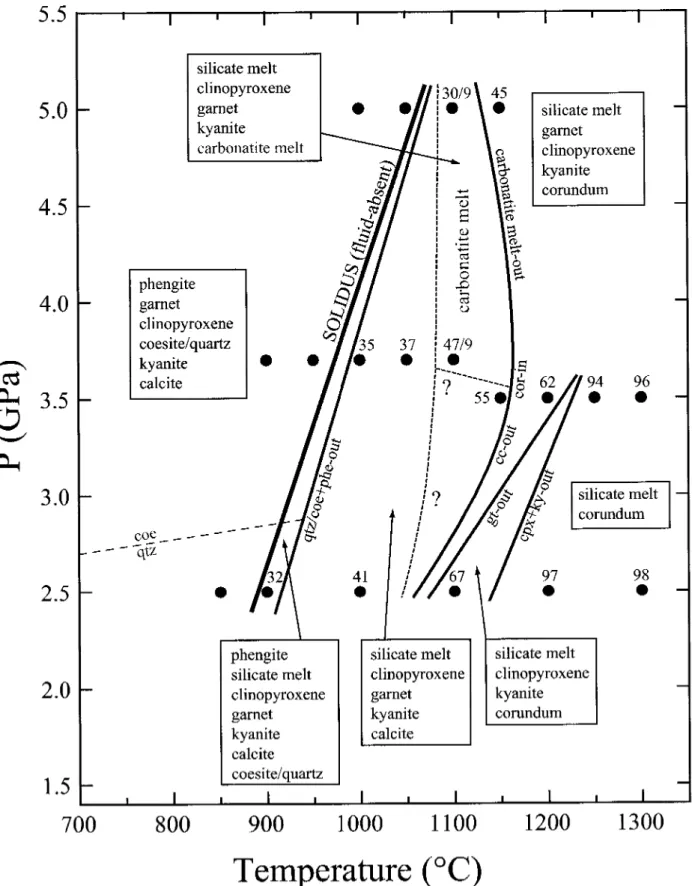
B, the carbonatite-phonolite liquid miscibility gap is depicted according to our experiments
C, 3.7 GPa, a total of three experiments were performed, two of which produced carbonatite fluid, while the third formed Fe-Mg calcite, indicating that these experiments are close to closure of the silicate+carbonate two-fluid solution. The convergence of reaction (1) with the Clapeyron slope of 70 °C/GPa to the nearly temperature-independent calcitetocarbonatite phase boundary (Fig. 5.1) further suggests that the carbonate melting reaction will exceed solid pressure in the absence of liquid than 5 without more. Therefore, other pressures, melting will start through carbonate melting, silicate melts will form only the highest temperatures, this is already the case > 2.5 GPa in K-free basal systems (YaxleyandBrey2004, Dasguptaetal, Dasguptaetal).
As the carbonatite melt compositions (Tab. 5.3B) imply, the generation of carbonatite in the current sedimentary and also in the basaltic systems (YaxleyandBrey2004,Daguptaetal.2005,2006) can be approximated in the (Fe,Mg,Ca.Ca.203) system. . .7 and 6.0 GPa (Irving and Wyllie 1975, Buobetal. 2006) for compositions with Xf.a=Ca/(Ca+Fe+Mg)=0.4 and 0.5, respectively. The carbonate-saturated pelitic lithologies of the subducting crust are composed of an eclogitic phengite -i-garnct+clinopyroxene+kyanitc J-Fc-Mg-calcitciquartz/coesitesubsolidus phase assemblage between 75 and 150 km deep. C02 is expected to be released, i.e. only <5 % of total C02, through metamorphic reactions, due to 0.05-0.15 of inmetamorphic fluids at low temperature, high pressure conditions (Kerrick&Connolly2001-sufficient 2ft0000,Connolly2001,2001 -2001-2001,2001-2001,2001-2001.0% of total C02 is flushed through decarbonization driven by infiltration with serpentine-released aqueous fluids below the sediment layer (Connolly2005) and extremely hot.
Nakamura2003).In the Northeast Greenland Eclogite Province (NEGEP) eclogitcs with biotites formed>1.5 GPa and 700-780 °Care rich in MgO and CaO but very poor A120, (Brueckner . etal. 199). Therefore, in this exploratory study, we experimentally investigate the high-pressure phase petrology of carbonate-saturated, Mg-rich (and Fc-frec) pelites, focusing on the stability of K-mica and biotite in particular.
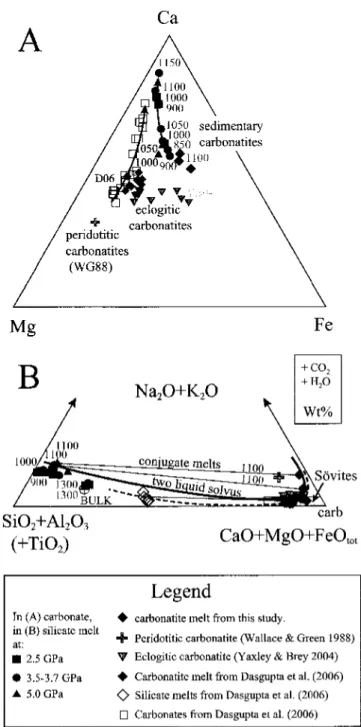
Experimental and analytical techniques
- Analytical techniques
The pressure was calibrated against the quartz coesite transformation at 3.1 GPa and 1000 °C (Bosc&Ganguly 1995), the fayalitctoy-spink reaction at 5.25 GPa and 1000 °C (Yagictal. 1987) and 100 °C, p. usakietal.1985). The capsules were centered on the hot spot within ± 0.7 mm, the thermocouple at the top of the piston cylinder experimented thus recording the coldest temperature in the capsule. Thermal gradients along the entire length of the capsules were evaluated at 10-20 °Cat 10050-10 °C and 1050 °C. The base of the starting material was synthetic glass made from oxides SiO2, Al2O3, MgO, CaO mixed with natural alkalifeldspar.
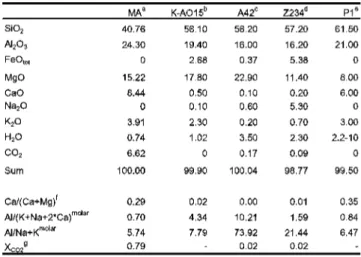
Experimental results
Non-ideality of biotites at high pressures
Between crystallochemical effects, deviation from ideal biotite solid solution to series of phengite solid solutions at high pressure occurs mainly through the coupled di-tri-octahedral substitution2VI(AP')+VI0<->3VI(Fe2+, Mg2+), resulting in .
The stability of carbonates compared to previous studies
Concluding remarks
Summary, On-going work and Outlook
Preliminary results
Calculated proportions (in vol%) of formica, amphibole, clinopyroxene and dolomite are shown in fig. 7.1D-G, which also fits surprisingly well with the calculated proportions (urban mass balance) from the experiments (see sec Tab.4.2). The conclusion is that this first attempt to implement a model of the subassembly.
Field study of carbonaceous pelites from high-pressure terrains
Permomesozoic carbonaceous and pelitic metasediments of the Penninic Range, south of the Gross Grossvenediger, TauernWindow, exhibit high. pressure Alpine phase assemblages with phengite overprinted by lower pressure biotite-bearing grccnschist-amphibolite facies assemblages. The phengite-biotite transforma¬. tial field in Dorfcrtal and Timmeltal, north of the Virgenthal. Naissance fieldwork combined with this study is not included in the thesis. Dachs E (1986) High-pressure mineral assemblages and their breakdown products in metasediments south of the Grossvenediger, TauernWindow, Austria. RiederM, CavanazziG, D'YakonovY, Frank-KamenetskiiVA, GottardiG, GuggenheimS, KovalPV, MüllerG, NievaAMR, RadoslovichEW, RobertJ-L, SassiFP, Takeda H, Weiss Z and Wones DR(1999) Nomenclature of the mica.
RiederM,CavanazziG,D'YakonovY,Frank-KamenetsknVA,GottardiG,GuggenheimS,KovalPV,MüllerG,NievaAMR, RadoslovichEW,RobertJ-L,Sassi FP,TakedaH,Weiss Z and Wones DR(1999)Micas Minaclature of the ¬. Shatsky V, SobolevNV, Vavilov MA(1995) Diamond-bearing metamorphicrocks of the Kokchetav Massif (northernKa¬. zakhstan) In: ColemanRG, WangX(eds) Ultrahighpressuremetamorphism. Sorensen, SS(1986) Petrological and geochemical comparison of blueschists and greenschists in the Catalina Schist terrane, Southern California Geological Society of America Memoir 164-59-75.
VielzeufD, Holloway JR (1988) Experimental determination of fluid-absent melt conditions in the pelitic system Implications for crustal differentiation. Contribution to mineralogy and petrology 98-257-276. ZhangRY, UtsumiW, Liebermann RC(1996) In-situ X-ray observations of the coesite-stishovite transition-reverse phase boundary and kinetics Physics and Chemistry of Minerals 23: 1-10.
CURRICULUM VITAE
August 1969
EDUCATION