I would like to thank Professor Emeritus Sotirios Ves, Special Teacher Triantafyllia Zorba and Dr. I would also like to thank the members of the Commission, Associate Professors Maria Katsikini and Joseph Kioseoglou (Aristotle University of Thessaloniki).
Introduction
Under this new context, a number of phenomena ranging from absorption and scattering of EM radiation, electrical and thermal conductivity to superconductivity can be understood as interactions of phonons with other fields.
Basic Approximations in Lattice Dynamics
The Adiabatic Approximation
Ξ(r, R) obeys the Schr¨odinger equation for the entire system (eq 1.2), if the ion wave function Ψ(R) obeys the modified Schr¨odinger equation. This expression can be easily derived by substituting Equation 1.4 into Equation 1.3 and using Eq.
The Harmonic Approximation
In the next section we will prove that, through appropriate transformation of variables, it can be expressed as a system of independent, non-interacting 3M oscillators. In this paper, we will only deal with the harmonic terms, since the material under study exhibits moderate anharmonicity.
Harmonic Approximation: The Eigenvalue Problem
The Force Constant Tensor
This tensor has some important properties that we will find more useful later, which derive from the symmetries of the crystal, namely the invariance of the potential against infinitesimal translations of the crystal as a whole, as well as against discrete translations of the crystal lattice . . 1.13, the second (eq. 1.17) is a result of the infinitesimal translation invariance and the third (eq. 1.18) arises from the discrete translation invariance of the HamiltonianHh.
The Periodic Boundary Condition
This is another expression for the periodic boundary condition in q-space and it is essentially the origin of the definition of the reciprocal q-space and of the Brillouin zone, the latter being a direct consequence of translational periodicity in q- space if so. Therefore, if |ni| > Ni/2 (the q-vector is outside the Brillouin zone), by an appropriate choice of the integers mi, we can carry the q-vector inside the Brillouin zone, to a new, equivalent q-vector with the same physical result.
Normal Coordinates and Diagonalization
Therefore, if we express Φab(lk;l0k0) = Φab(l−l0, k; 0k0) ≡ Φab(h;k0k0) and replace the summation over l enl0 with a summation over h enl0, and use the lattice sums, we get the new form of the Hamiltonian 1.35. We will prove below that j refers to the set of eigenfrequencies corresponding to each q-value and that thea(k|~qj),a= 1,2,3 are the corresponding eigenvectors.
The Dynamical Matrix
This relation can be easily proven by simply applying the definition of reciprocal space vectors (eq. 1.24). Also, the polarization vectors of the 3n-dimensional mode are transformed according to some 3n-dimensional representation of the array.
Quantum Mechanics
Finally, the initial (u, p) operator pair can be expressed in terms of the learning operators by substituting 1.61 and 1.62 into 1.49. Thus by expressing those operators in terms of the learning operators and expressing the eigenstates as generalized Fock states 1.67, the calculations become considerably easier.
Density of States and Connection with Thermodynamics
Then the number of these states is equal to the ratio of the volume Vω of the shell between the constant energy surfaces of Figures 1.3a and 1.3b over the volume occupied by a single q-point Vq, assuming of course that we have about a macroscopic system, for which Vω →0. This is of course a complicated surface integral, and to calculate it we need complete knowledge of the constant energy surfaces in the entire Brillouin zone, which is very difficult to obtain.
Structure
This implies that there is an asymmetric unit inside the unit cell which, if acted upon by all 48 symmetry operations of the space group, reproduces the entire unit cell. The Wyckoff positions of each atomic species within the asymmetric unit are summarized in table 2.1. The crystallographic unit cell therefore consists of a complex network of coordination polyhedra (octahedrons, tetrahedra and dodecahedra) which are translated and rotated according to the symmetry requirements of the space group.
Atoms within the unit cell that belong to incomplete polyhedra (that are not completely contained in the unit cell) are also shown. So we can say that smaller coordination numbers in YAG lead to shorter bond lengths. These polyhedra form another structural subunit of the lattice cell, containing a total of 23 atoms.
The polyhedra within this subunit are positioned in fixed relations to each other, therefore the entire subunit is repeated as a whole, through a series of rotations and translations that make up the space group, thus reproducing the crystal.
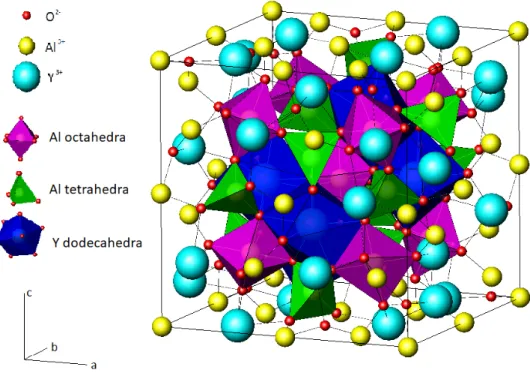
Phenomenological Models and the Shell Model
Calculation of the Raman Tensor in the RIM gives exactly zero, while experimentally measured Raman active modes are clearly present (see Chapter 4). This is an extension of the RIM, which takes into account the electronic polarizability in a very simplistic way. It also has zero mass, which matches the requirements of the Adiabatic Approximation, as we discussed in the previous chapter.
There is also an extended form of the Shell Model due to Cochran et al. 6], in which short-range core-core and core-shell interactions are allowed. In this work, we make use of the Shell Model to describe the interatomic forces within YAG. Therefore, in a good implementation of the model, the parameters are set in such a way that internuclear distances do not fall below the threshold of infinite attraction.
Also, the parameters used for the Born-Mayer part of the Buckingham 2.3 potential were among those reported by Milanese et al.
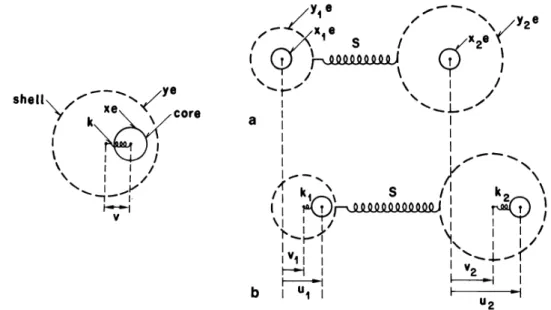
The Quasi-Harmonic Approximation
In this work, we will treat anharmonicity by means of the quasi-harmonic approach, which is valid for weak anharmonic effects. We will now derive the thermal dependencies of the fundamental natural frequency ω =ω(T) and of the average equilibrium distance a(T), where a =< r >. The expansion of the potential around the initial equilibrium a0 with terms up to third order was given in 2.6.
The new force constants can be expressed in terms of the old ones by entering the deformation (T, p) as. It can also be shown that the first-order term eu in the potential is of the same order of magnitude as that of the third order. The core assumption of the quasi-harmonic approach is that the anharmonic part Aanh is neglected and thus A≈Aqh.
The quasi-harmonic term neglects the anharmonic terms of the interaction potential, which means that the HamiltonianHqh.
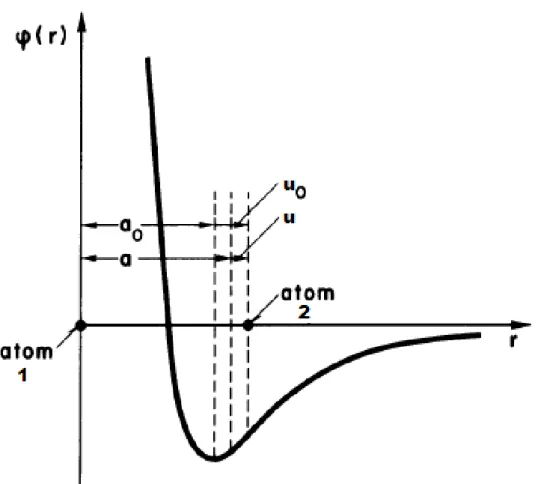
Calculations with GULP
- Static Calculations
- Dynamical Matrix
- Density of States
- Free Energy Minimization
A final issue that needs to be properly addressed is that of the shell coordinates that appear in the expression of the force constant matrix. Therefore, it should only contain the degrees of freedom belonging to the ionic cores within the unit cell, not those in the shells. Instead, a numerical evaluation is performed using the q-grid method, also known as the Monkhorst-Pack scheme [28].
However, GULP by default uses part of the symmetry of the Patterson group to reduce this number. However, in practice, the Patterson group symmetry of the crystal reduces the number of independent, non-degenerate points to 52. The total internal and free energy is simply the sum of the vibrational and static parts (equation 2.34).
Elastic constants represent other derivatives of the energy density with respect to strain (see J.F. Nye [30]).
Results and Discussion
- Lattice Constant and Thermal Expansion
- One-phonon Density of States
- Heat Capacities
- Entropy
- Free Energy
- Elastic Properties
- Gr¨ uneisen parameter and anharmonicity
That is, the low-energy phonon modes are attributed almost entirely to vibrations of the yttrium atoms, as well as to vibrations of molecular units as a whole. Finally, our calculations agree well with the results of inelastic neutron scattering measurements of the density of states of the material (unpublished work by K. Papagelis). In Figure 1.6 we present the measured values of the total density of states, compared with our calculations.
Considering the complexity of the structure and the calculations themselves, the agreement seems very satisfactory, while the data agree with the existence of the mentioned energy gap in the region of around 650 cm−1. The current version of the code contains the output of the Helmholtz free energy of the equilibrium state, as well as its components, namely the static and vibrational parts. Figure 1.11 also shows the components of free energy, namely the short-range potential, electrostatic and vibrational parts.
Where our model fails, however, is to predict the general downward trend of the anisotropy ratio with increasing temperature (Figure 1.16).
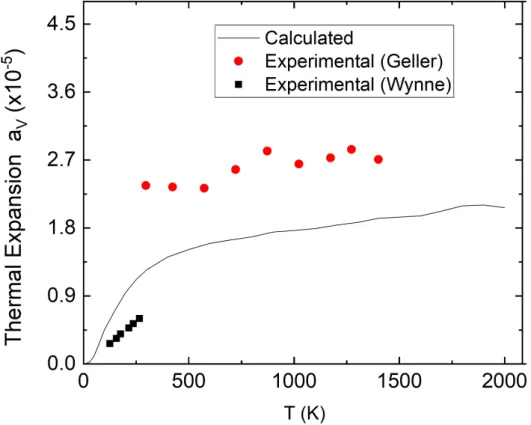
Raman Scattering
If we consider only terms up to the first order in E, we have normal Raman scattering. If we include higher-order terms, we are dealing with the hyper-Raman effect; under conditions. As for normal scattering, it is evident that the electronic polarizability strongly depends on the nuclear configuration.
Due to thermal fluctuations (see Chapter 1), the system is in a distorted configuration that also depends on time. Recalling from Section 1.4 that the normal coordinates completely diagonalize the harmonic Hamiltonian, it is easy to show that, using the classical equations of motion, the time dependence of Qs can simply be written as Then, by combining equations 1.1–1.7, after a long series of substitutions, trigonometric identities, and time-averaged integrations, we can show [5] that the average intensity radiated in the k direction is the same.
The first term is the Rayleigh scattering term, while the (ωL±ωs)4 are the Stokes(-) and anti-Stokes(+) components, respectively.
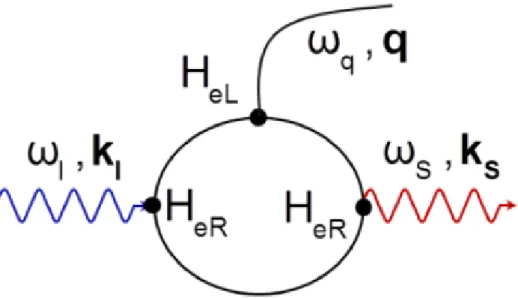
Results and discussion
Therefore, we can determine the Raman active modes simply based on the values of the Raman Intensity Isc calculated directly by GULP for each mode:. In the rest of this chapter we will focus on the study of the calculated phonon polarization eigenvectors for some Raman active modes. For this reason, only the eigenvectors associated with the structural subunit of the unit cell shown in figure 2.3 are depicted.
This means that analysis in terms of bond bends, stretches and molecular vibrations of coordination polyhedra is possible. With the exception of the high-frequency mode, they are in excellent agreement with previous studies [31] using the RIM model. The vibrations of the tetrahedral and octahedral units for both groups are clearly visible in Figures 4.6 and 4.7.
As expected, the low-frequency mode ω4 = 153cm−1 (Fig. 4.8) is mainly due to vibrations of the heavy Y ion, as well as small translational and rotational movements of the Al−O polyhedra.
![Figure 4.2: Raman spectrum of YAG at ambient conditions reported by [3].](https://thumb-eu.123doks.com/thumbv2/pdfplayerco/302307.42101/77.892.129.790.292.669/figure-4-raman-spectrum-yag-ambient-conditions-reported.webp)