PSEBS polystyrene-block-poly(ethylene-ran-butylene)-block-polystyrene PSEBS CM chloromethylated polystyrene-block-poly(ethylene-ran-butylene)-. The first group is focused on the preparation and characterization of ion exchange membranes, while the second group is on the preparation and characterization of ionic liquids.
Membranes – general overview
Types of membranes
Composite membranes – the newest types of membranes belong to the group of multilayer composite materials. Inorganic materials - ceramics, metals (eg, steel, palladium, gold) glass (SiO2), oxides (eg, Al2O3, ZrO2) - precedents of these membranes are chemical and thermal resistance and narrow pore size distribution.
Basic transport characteristic of the membranes
On the other hand, the value determines the amount of the freely moving ion carriers in the membrane, which are available for the charge transport in electric field. According to these facts, the ion exchange capacity greatly affects the ion conductivity of the membrane (5). c) Ionic conductivity – it is possible to determine by several procedures.
Ionic liquids
The second option is based on the arrangement of the electrodes, divided into systems with two or four electrodes. On the other hand, with an addition of lithium salt, this fact can increase viscosity, which can lead to a decrease in electrolyte conductivity (13).
Electro-membrane´s processes
- Fuel cells
- Alkaline water electrolysis
- Microbial electrolysis
- Lithium-ion batteries
Polymer electrolyte fuel cell (PEFC) - the electrolyte is an ion exchange membrane, which is an excellent conductor of ions. Molten carbonate fuel cell (MFCF) - the electrolyte is usually a combination of alkali carbonates, which is held in a ceramic matrix of LiAlO2.
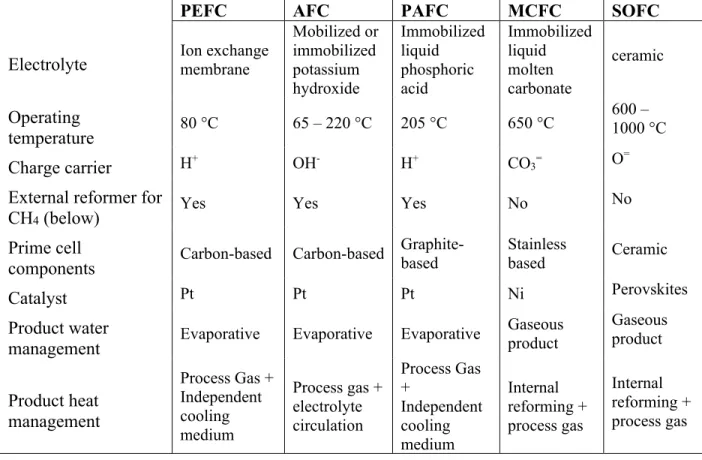
Materials
Chemicals for PSEBS-CM-TMA synthesis
Chemicals for VPA-co-AN synthesis
Chemicals for PSEBS SU synthesis
Chemicals for preparation of Ionic Liquids
Synthesis, polymerization and preparation
- polystyrene-block-poly(ethylene-ran-butylene)-block-polystyrene (PSEBS)
- Poly(vinylphosphonic acid-co-acrylonitrile) synthesis (VPA-AN)
- Preparation of sulfomethylated polystyrene-block-poly(ethylene-ran-butylene)-
- Preparation of ionic liquids and electrolytes
Membranes were cast from a 5% PSEBS-CM solution in chloroform, dichloromethane, toluene, or tetrahydrofuran on a Teflon plate. PSEBS-CM quaternization was performed by immersing a PSEBS-CM membrane with a degree of chloromethylation of 54% in an excess of TMA solution for 48 h at room temperature. Commercial PSEBS and uncrosslinked chloromethylated PSEBS-CM (Table 4) are well soluble at room temperature in nonpolar or slightly polar solvents, such as cyclohexane, toluene, tetrahydrofuran, diethyl ether, tetrachloromethane, chloroform, and dichloromethane.
On the other hand, no solvent was found that dissolves PSEBS-C-TMA in the form of a membrane or powder. The polymers were precipitated from the reaction mixtures in ethanol, filtered and washed thoroughly with ethanol. The concentration of VPA units in the polymer increases with increasing concentration of VPA monomer in the polymerization mixture (which is plausible), AN is more easily incorporated into the polymer chain than VPA.
A partial hydrolysis of AN groups in the polymer in the presence of VPA can be assumed, it is well known that nitrile groups are subject to hydrolysis in an acidic environment (42). After this time, the reaction mixture was cooled to room temperature and a viscous yellowish product was obtained. Methyl iodide (43 g, 0.3 mol) was added with stirring at room temperature (over a period of 1 h) to tributylphosphine (61 g, 0.3 mol) dissolved in methanol (150 mL) in a 250 mL three-necked flask equipped with a thermometer and reflux.
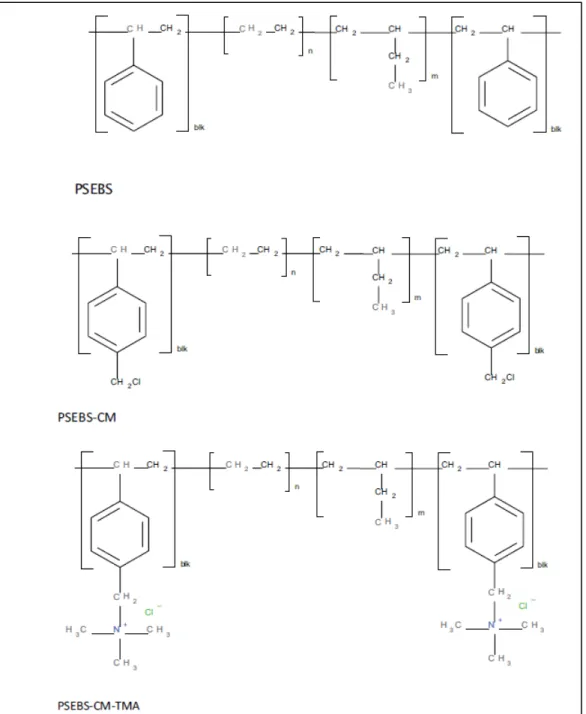
Techniques
- Molecular weight determination
- Elemental analysis
- Fourier-transform infrared spectroscopy (FTIR)
- Nuclear magnetic resonance (NMR)
- X-ray fluorescence (XRF)
- Small-angle X-ray scattering (SAXS)
- Thermogravimetric analysis (TGA)
- Differential scanning calorimetry (DSC)
- Water uptake (WU)
- Oxidative stability
- Contact angle (CA)
- Transport number and permselectivity
- Ionic conductivity (IC)
- Ion exchange capacity (IEC)
- Cyclic voltammetry
Glass transition temperature (Tg) was determined from the heating run as the midpoint between the glassy and rubbery branches of the DSC trace. After the dried membranes were measured, the membranes were immersed in demineralized water at 25, 60 and 90°C, respectively, until they reached a constant weight (24 hours). A membrane was thoroughly washed in water and then dried and weighed, then the membrane was re-swollen in water (48 hours), then immersed in Fenton reagent (0.5 g of the membrane in 25 g of the Fenton reagent) for 1 hour at 70˚C and dried and weighed again.
To measure CA, the sitting drop method was used with an optical contact meter (OCA20 DataPhysics, version 2.03) equipped with a CCD video camera with a resolution of 768 × 576 pixels and up to 50 frames per second and an integrated temperature measurement unit TC400 and a display in the range of -40 to 400 °C. The membranes were placed in a two-chamber measuring cell (each chamber had a volume of 25 ml with an Ag/AgCl reference electrode facing the membrane surface from both sides) and the chambers were filled with 0.5 M and 0.1 M KCl solution, respectively. Permeselectivity was calculated by considering Em and the theoretical potential difference in a fully permelectic membrane (Eth) as described elsewhere (43).
The in-plane IC of the membranes was determined in a tempered box in a gas-tight cell in a deionized water environment using a four-electrode arrangement for electrochemical impedance spectroscopy. The ion exchange capacity (IEC) was measured by potentiometry during the transition of the membrane sample from the OH- to the Cl- form. The potential value of the glass electrode was converted into the concentration of displaced OH- ions in the solution using a calibration curve.
Characterization of prepared materials
- Quaternized chloromethylated polystyrene-block-poly(ethylene-ran-butylene)-
- Poly(vinylphosphonic acid-co-acrylonitrile) membrane
- polystyrene-block-poly(ethylene-ran-butylene)-block-polystyrene
- Ionic liquids
Elemental analysis was used to confirm successful sulfomethylation on PSEBS-CM membrane, three runs of the sample were performed, values are summarized in Table 11. According to the calculation, it was confirmed that chloromethylated conversion of the membrane was about 54%, resulting in 2.56% content of sulfur recorded by elemental analysis. These results confirmed very good transport and permselectivity properties of the membrane as well as the stability of the membrane in microbial environment.
31P NMR spectra of those prepared ionic liquids were shown in the second part (1b, 2b, 3b) of the figure. The triplet doublet observed at Part 1b appeared as a result of the pulse program of the experiment. The effects of the addition of Li+ salts in ILs on the thermal behavior of the prepared mixtures were studied by differential scanning calorimetry.
However, DSC measurements of their mixtures with Li+ salts revealed ionic interactions that caused amorphization of the IL matrices. The ionic conductivity of the prepared ILs with different types of additional lithium salts at different concentrations are shown in Table 12. EtMeIm]+ [EtPITE]- shows poor conductivity properties with any of the Li salts used due to its high viscosity.
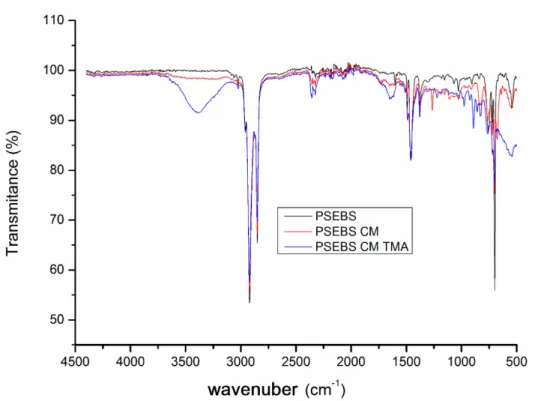
Application
Fuel cell test
Alkaline water electrolysis
As a separator of the anode and cathode space, heterogeneous anion selective membrane under load curve measurement or a PSEBS-CM-TMA membrane prepared in this work in a long-term experiment was used. The scheme of the electrolysis process with the role of the anion-selective membrane can be seen in Figure 24. The influence of the amount of PSEBS-CM-TMA in the catalytic layer (anodic) was also tested.
The rationale for better catalyst utilization when using the PSEBS-CM-TMA polymer binder instead of the PTFE binder lies in the nature of both materials. The contact with the reactant is easily achieved due to the presence of the liquid water in the system. On the other hand, this is related to the lower flexibility of the water electrolyzer and the higher safety risk.
The other option could be the use of the anion-selective material as a polymer binder. Such a material should be able to ensure the ionic conductivity even in the environment of the dilute liquid electrolyte. The long-term stability of PSEBS-CM-TMA in an alkaline environment was tested in a laboratory electrolyzer, where the PSEBS-CM-TMA membrane was used as a separator of the anode and cathode compartments.
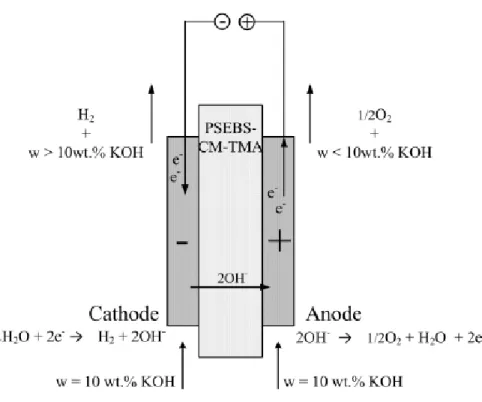
Microbial electrolysis cell (MEC)
The CF22 R14 qualified in 3rd place, while the FBM finished at the bottom of the line again. It can be assumed that either the higher mass transfer of propionate and butyrate – and the associated consequences – is the source of the lower score in the case of VFA mixture, or that the mass transfer of VFA in general increased more and more during the operation period due to change in membrane stability. The output of the MEC is a function of, among other things, the composition of electrolytes and the transfer of ions between anolyte and catholyte that occurs through the applied membrane (60).
Therefore, the evaluation of the membrane performance in terms of ion transfer can be of great importance. The final pH of the catholyte for both CEMs reached 12.8–13.0 due to the enrichment of OH- ions in the cathode chamber. The catholyte conductivities in CEM-equipped LEC showed increasing trends across the groups (Table 15), especially in the case of PSEBS SU22-MEC (e.g., catholyte conductivities of mS cm-1 and mS cm-1 were observed with acetate and VFA mixture, respectively).
However, the use of the FBM membrane in MEC resulted in a lower pH imbalance (ΔpH) than in the case of CEMs. Cathode surplus potentials for FBM-MEC were significantly higher in the (-2.0 V regardless of substrate), nearly twice that obtained for CEMs (Table 16). The high cathode overpotential in the case of FBM-MEC is an apt example: the reduced pH imbalance due to the use of a bipolar membrane (and the associated migration of protons and hydroxide ions from water splitting in the transition region of FBM) does not compensate for the additional losses due to the functional and ion transport character (64), (58).
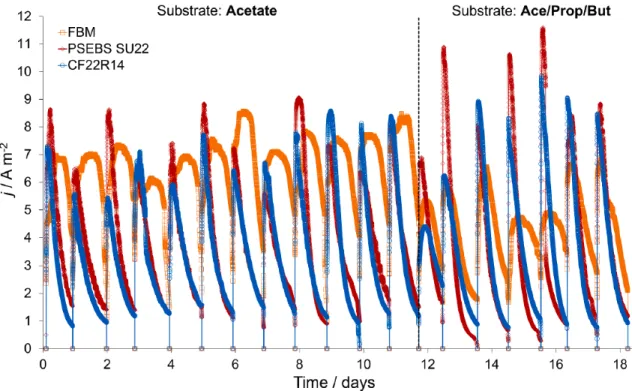
Coin cell test
This ionic liquid can be used as an electrolyte in lithium-ion batteries in case additional SEI resources are used. With further development, this IL can also be used as an electrolyte in lithium-ion batteries. Feasibility of quaternary ammonium and 1,4-diazabicyclo[2.2.2]-octane functionalized anion exchange membranes for biohydrogen production in microbial electrolysis cells.
Chapter One - Electrolytes for Li- and Na-Ion batteries: concepts, candidates and the role of nanotechnology. Anion exchange membranes and binders based on polystyrene-block-poly(ethylene-ran-butylene) block-polystyrene copolymer for alkaline water electrolysis, desalination and water treatment. Bouzek: Ion exchange membranes based on copolymers of vinylphosphonic acid and co-acrylonitrile for fuel cells, desalination and water treatment.
Konefal: Synthesis and electrochemical properties of electrolytes based on phosphonium, sulfonium and imidazole ionic liquids for Li-ion batteries, 2023. Bystroň: Polymerizable vinylimidazole-based ionic liquids for Li-ion batteries, International Conference on MembranesFive and Environmental Processes20Elect. polymer gel electrolyte for lithium-ion batteries based on polyphosphazene, Visegrad Membrane Conference - PERMEA and International Conference on Membrane and Electromembrane Processes - MELPRO.
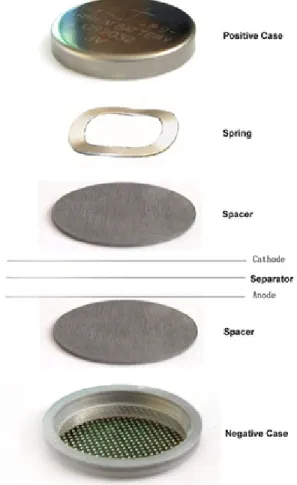