Application of molecular tools for detection of plant viruses
Texto
Imagem
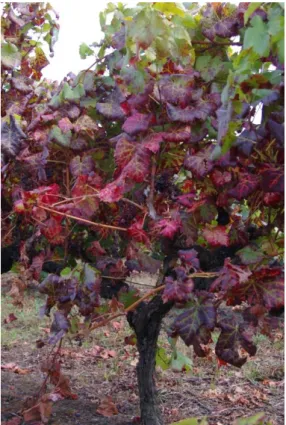
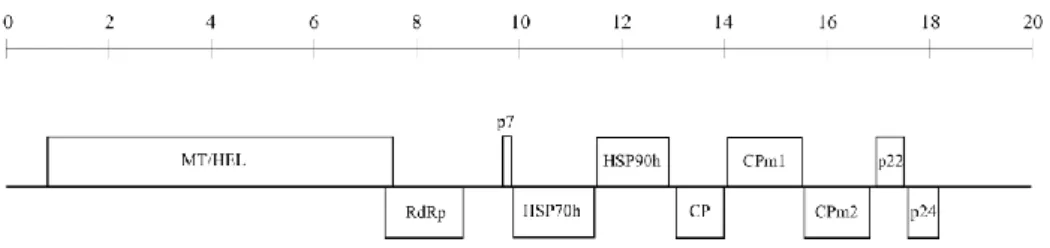
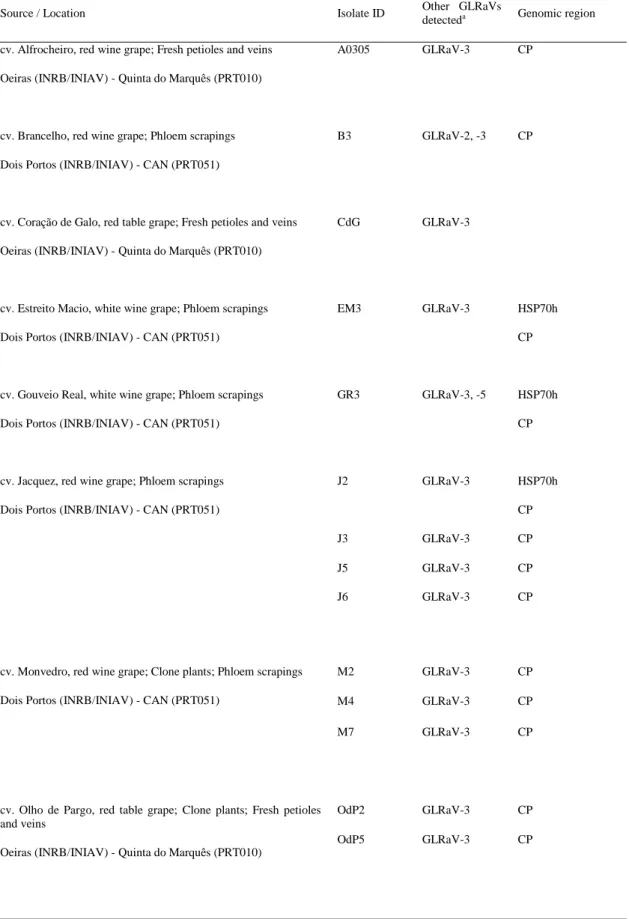

Documentos relacionados
Figure 29 – Theoretical results for the FWM signal without absorption for three intensities of the input lasers using the complete solution (blue) and only the direct pathway
Foi utilizado o conceito Lean Canvas (Maurya, 2012) como modelo de negócios de forma a expor o projecto, e também as metodologias Lean para eliminar recursos e actividades
The probability of attending school four our group of interest in this region increased by 6.5 percentage points after the expansion of the Bolsa Família program in 2007 and
No Comité de Embaixadores foram adiantados os primeiro contornos de algumas propostas que poderiam começar a concretizar-se: o enquadramento sistemático na CPLP
ções às escolas para a implantação de Programas de Saú- de. Deve possuir conhecimentos referentes à Educação da Saúde e estar <Jpto a desenvolver uções
The objective of this study was to model, analyze and compare the structure of spatial dependence, as well as the spatial variability of the water depths applied by a
The nurses agreed with the universal access to technology, which should also consider the results of their use and the professional opinion based on what is best for the