82 IV-B Determination of hydroxyl radicals from photolysis of Fe(III)-carboxylate complexes in aqueous solutions. B-1-2 Effect of pH on the formation of hydroxyl radicals in the irradiated aqueous solution containing Fe(III)-Pyr complexes……. B-1-3 Effect of Fe(III) and Pyr concentrations on the photogeneration of hydroxyl radicals in the aqueous solution……….
B-2-2 Effect of pH on the formation of hydroxyl radicals in the irradiated aqueous solution containing Fe(III)-Cit complexes……. B-3-2 Effect of pH on the formation of hydroxyl radicals in the irradiated aqueous solution containing Fe(III)-Tar complexes……. B-3-5 Effect of temperature on the formation of hydroxyl radicals in the irradiated aqueous solution containing Fe(III)-Tar complexes…….
INTRODUCTION
Until now, 2,4-D and its related product 2,4-DCP are very popular pollutants in the organochlorine family. Advanced oxidation technologies are believed to be the preferred means of degrading 2,4-D and 2,4-DCP. The aim of this work is to understand the fate of pollutants in the aquatic environment in the presence of light and various organic complexes of Fe(III) naturally present in the aquatic environment.
In this work, we first studied the physicochemical properties of Fe(III)-carboxylate complexes, including complexes of Fe(III) with citric acid (Cit), tartaric acid (Tar) or pyruvic acid (Pyr). Second, the photogeneration of ·OH and peroxyl radicals was determined in aqueous solutions with different iron carboxylate complexes. In this study, the quantum yields of Fe(II) formation, the disappearance of 2,4-D and 2,4-DCP were determined.
BIBLIOGRAPHY STUDY
Iron in the environment and its photochemical properties
In the aqueous solutions, Fe(III) is not stable and usually exists in the form of Fe(III)-hydroxy complexes. On the other hand, pH affects the distribution of Fe(III) species in the aqueous solutions. Photolysis of Fe(III)-Carboxylate complexes can form Fe(II) and generate reactive substances which quickly react with the pollution, so the reaction will continue in such a way that Fe(III) is reduced to Fe(II).
Lower concentration of Fe(II) in the solutions, lower formation of radicals, thus the photodegradation efficiency of 2,4-DCP is low. As shown in Figure IV-C-27, the concentration of Fe(II) reaches 80% of the total iron in the solutions after 10 minutes of irradiation. In the second step, the concentration of Fe(II) was stable from 2 hours to 5 hours of irradiation.
It differs from the results obtained in the presence of Fe(III)-Tar complexes for a long irradiation time, where the degradation of 2,4-DCP decreased for a higher concentration of Fe(III)-Tar complexes. In the presence of 0.3 mmol.L-1 Fe(III)-Pyr, iron was completely transformed into Fe(II) after 45 minutes of irradiation. Oxygen can enhance the photodegradation of 2,4-DCP in the presence of the Fe(III)-Pyr complex.
In deaerated solution, the quantum yield of 2,4-D is negligible, especially in the presence of Fe(III)-Cit and Fe(III)-Tar complexes. Effects of 2,4-D concentration on the quantum yields of Fe(II) and 2,4-D were studied in the solutions containing 0.3 mmol.L-1 Fe(III)-carboxylate complexes (Fe(III)-Cit , Fe(III)-Pyr and Fe(III)-Tar). The photochemical properties of Fe(III)-carboxylate complexes have been studied systemically in the presence of 2,4-D.
In the presence of the Fe-Pyr complex, the concentration of 2,4-DCP is higher than other complexes.
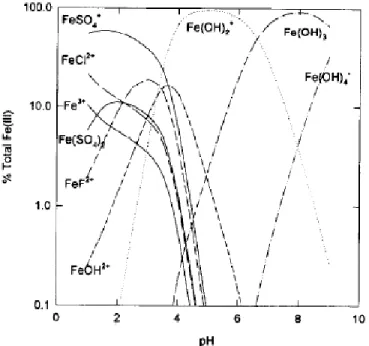
The Iron-Carboxylate complexes
Chlorophenols
The Environmental Protection Agency (EPA) was notified of the death of a worker acutely exposed to 2,4-DCP. 2,4-DCP was also reported to be found in the leachate from an industrial landfill (Brown et al., 1988). The environmental fate and transport of 2,4-DCP is controlled by its physical and chemical properties and environmental conditions.
Evaporation of 2,4-DCP from water is expected to be slow and therefore not a major removal process from surface water. The biological treatment of wastes containing 2,4-DCP has shown that neither chemical is removed by stripping (Stover and Kincannon, 1983). Thus, the environmental fate and transport of 2,4-DCP tends to partition into sediments and lipids and to bioconcentrate.
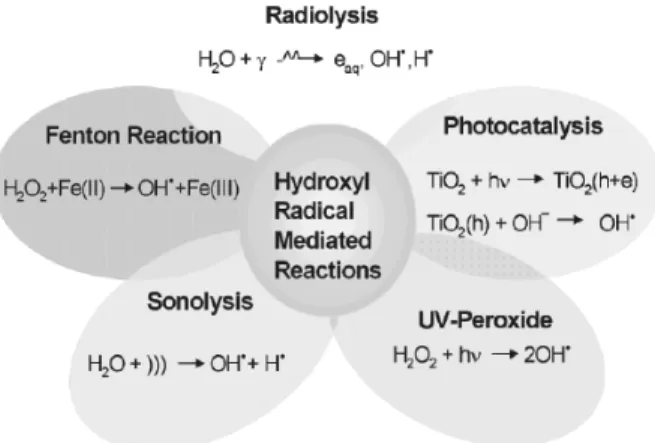
The 2, 4-Dichlorophenoxyacetic acid (2, 4-D)
2,4-D, MCPA, and mecoprop-p (MCPP-p) are chlorophenoxy acids developed in the 1940s and 1950s as growth-regulating herbicides for use against broadleaf weeds. Since its introduction in Canada in the 1940s, 2,4-D has become one of the most widely used and studied herbicides for use in agriculture, forestry, non-crop vegetation management, turf grass, lawn care and weed resistance management. Low concentrations have also been detected in surface water and drinking water in the US.
An increasing interest in the use of alternative processes for pesticide oxidation is registered in the literature. Other photocatalysis involves various iron-mediated oxidation systems, and the application of ferrioxalate-exchanged resin to the removal of 2,4-D in the presence of hydrogen peroxide and UV light has been studied (Kwan and Chu. It includes the formation of 2, 4) -DCR by attack of hydroxyl radicals to the ring and formation of 2,4-DCP by attack on the side chain.
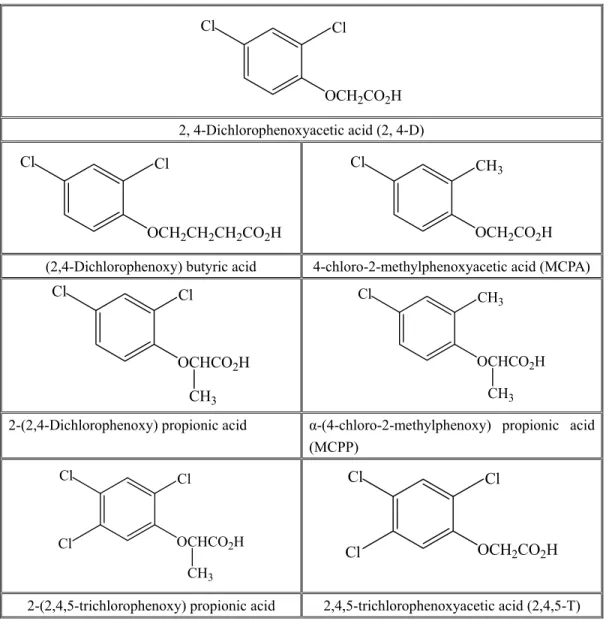
REAGENTS
PREPARATION OF SOLUTIONS
0.0815 g of 2,4-dichlorophenol was diluted to 500 mL by adding an appropriate volume of Milli-Q water to obtain the desired concentration of 2,4-dichlorophenol. The sodium vinegar buffer was prepared by mixing 600 ml of sodium vinegar (1 N) and 360 ml of sulfuric acid (1 N) with a final volume of 1 liter by adding an appropriate volume of Milli-Q water. Potassium ferrioxalate used for actinometry was prepared from potassium oxalate and ferric chloride, according to the procedure suggested by Calvert and Pitts (Calvert et al., 1966), and carefully stored in the dark.
These reaction solutions were freshly prepared from Fe(ClO4)3·9H2O stock or by diluting an aqueous stock solution of 2 mmol L-1 Fe(ClO4)3 to appropriate concentrations. If necessary, the reaction solutions were deaerated or oxygenated by flushing with argon or oxygen before irradiation. The cleaning time depends on the volume of the solution: 20 minutes for solutions with a large volume (100 ml) and 10 minutes for solutions with a small volume (5 ml).
IRRADIATION
The photon flux of the monochromatic irradiation at different wavelengths is listed in Table III-C-1. Where (1-10-ODλirr) represents the percentage of light absorption by the solution when t = 0, I0 is the number of photons entering the reaction cell per second, determined by actinometry, and l is the length of the irradiation cell in cm. L is the length of the cell used to measure optical density (OD).
These bands were used to calculate the yield of •OH produced in the system. The fraction of light absorbed at larger irradiation wavelengths and the intensities of light incident on the reaction volume are shown in Table III-C-2. A metal halide lamp was placed in the center of the plate, cooling water was used to maintain a constant temperature of approximately 25 ± 1 °C during the photoreaction.
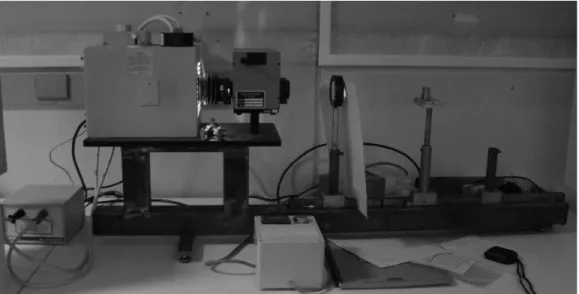
ANALYSIS METHODS
A Study of Physicochemical properties of Fe(III)-Carboxylate
B-2-3 Effect of Fe(III) and Cit concentrations on the formation of hydroxyl radicals in the irradiated aqueous solution containing Fe(III)-Cit complexes. B-3-4 Effect of Fe(III) concentration on the formation of hydroxyl radicals in the irradiated aqueous solution containing Fe(III)-tar complexes. The quantum yields of Fe(II) formation were studied in the aqueous solutions containing different Fe(III)-carboxylate complexes, namely Fe(III)-LTar, Fe(III)-DTar, Fe(III)-Pyr and Fe(III ). )-Cit complexes.
The quantum yields of Fe(II) generation were strongly influenced by the concentration of Fe(III)-carboxylate complexes. This decrease in Fe(II) can be explained by the reactivity of the OH radical on Fe(II). The quantum yields of Fe(II) formation are shown in Figure IV-D-5, which shows a tendency that with the increase of 2,4-D concentration the ΦFe(II) increases.
The values of ΦFe(II) are in the following order Fe(III)-Pyr > Fe(III)-Cit ~ Fe(III)-Tar.
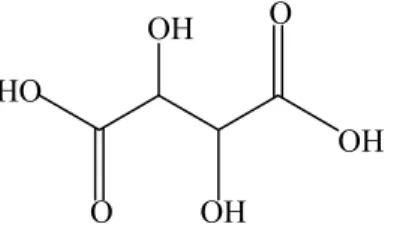
E-Photodegradation mechanism
E-1-Study of Fe(III)-Carboxylate complexes by ESR (electron spin
Irradiation experiments were performed at two different wavelengths λ ≥ 280 nm and λ ≥ 350 nm in the solution with Fe(III)-Cit complexes. As shown in Figures IV-E-3 and IV-E-4, the same ESR spectra signals were obtained in the presence of Fe(III)-Tar and Fe(III)-Pyr complexes. However, in the present study, there are no ESR signals indicating the formation of radicals for an initial pH = 6.0 in the aqueous solutions with Fe(III)-Tar and Fe(III)-Pyr complex under irradiation at λ ≥ 280nm (Figure IV-E-5).
In this work, both iron-carboxylate complexes and organic ligands can efficiently quench DMPO/·OH. In addition, in our previous work, we confirmed and determined the formation of hydroxyl radicals using benzene as a scavenger in aqueous solutions with iron-carboxylate complexes upon irradiation. The photodegradation of 2,4-DCP and 2,4-D in the presence of three types of Fe(III)-carboxylate complexes was investigated in previous parts of this work.
2,4-DCP is the most abundant primary photoproduct during the photodegradation of 2,4-D in the presence of Fe(III) complexes. No hydroxylated products of 2,4-D were observed by mass spectrometry. Figure IV-E-7 shows the kinetics of the formation of 2,4-DCP, the main photoproduct of 2,4-D, in the three different systems, Fe(III)-Pyr, Fe(III)-Tar and Fe(III)-Cit. This observation can be explained by the higher quantum yield of the disappearance of 2,4-D (0.046) than the quantum yield of the disappearance of 2,4-DCP (0.027) in the presence of Fe(III)-Pyr.
In the presence of Fe(III)-Tar, the highest concentration of 2,4-DCP is reached after 3 hours of irradiation and after a rapid decrease. This is consistent with the above 2,4-D degradation results that 2,4-D almost disappeared after 3 hours of irradiation. In the presence of Fe(III)-Cit, a surprisingly constant concentration is reached after about 1 hour of irradiation. According to the results in Figure IV-E-6, the degradation efficiency of 2,4-D is relatively low in the presence of Fe(III)-Cit than the other two Fe complexes.
In order to study the photodegradation process of 2,4-DCP, long-term irradiation was carried out in an aqueous solution of the Fe(III)-Tar complex at pH 3.0.
GENERAL CONCLUSIONS
APPENDIX