Electrospray ionization mass spectrometry as a valuable tool in the characterization of novel primaquine peptidomimetic derivatives
Texto
(2) 628. ESI-MS Characterization of Novel Primaquine Peptidomimetic Derivatives. action of 8-AQ against sexual stages of the malaria parasite also makes them the drugs of choice for malaria-transmissionblocking activity.3 Primaquine (1, PQ), however, cannot be used in patients having glucose-6-phosphate dehydrogenase deficiency as it causes hemolytic anemia4 and is affected by low oral bioavailability mainly due to its extensive conversion into inactive carboxyprimaquine, by oxidative deamination.5,6 Previous research devoted to minimize these problems was based on PQ acylation with amino acids and oligopeptides, which led to some progress in the drug’s therapeutic profile.7–13 However, amino acids and peptide derivatives of PQ are rapidly hydrolyzed to PQ by aminopeptidases and endopeptidases,10,12 suggesting that they might undergo extensive hydrolysis to the parent drug in the GI tract when given orally. Over the last five years, we have been working on peptidestable derivatives of PQ obtained by acylation of its primary amino group with an amino acid to yield a-aminoamides such as 2 or 3, which are then converted into imidazolidin-4-ones such as 4 or 5 by reaction with a carbonyl compound.14–17 Some of these imidazolidin-4-ones were found to inhibit the development of the sporogonic cycle of Plasmodium berghei malaria as efficiently as the parent drug,15 while being highly stable both in human plasma and isotonic buffer.14,15 Further, these compounds were recently found to be active against both Pneumocystis carinii and the chloroquine-resistant Plasmodium falciparum W2 strain, in the µM range.18 Taking 4 and 5 as leads, we set out to prepare peptido mimetic derivatives of PQ 6–8, whose structures can be regarded as mimetics of PQXaaPro/PQProXaa a-aminoamides such as 3.19,20 Compounds 8 were recently found to be highly stable at physiological pH and T, while being active against the Plasmodium falciparum W2 strain and efficiently inhibiting the development of the sporogonic cycle of Plasmodium berghei.20 In turn, compounds 6 and 7 were found to be potentially useful as PQ prodrugs.19 Given the relevance of compounds 4–8 as prospective pharmaceuticals, we have recently developed a methodology for further physico–chemical characterization of the title compounds by means of ESI-MS techniques.21,22 Our aim was to get a deeper insight into the structural features of drug-derived structures bearing both the imidazolidin-4-one and the 8-AQ scaffolds, as similar studies are scarce in the literature. Moreover, the most relevant examples of analytical studies focused on PQ and related structures23–41 are essentially focused on compound separation/identification, with very few examples where MS fragmentation studies are the core of the reported work. Therefore, the present is a brief report on the comparative study of the aforementioned families of PQ-derived imidazolidin-4-ones (4–8) by ESI-MS fragmentation techniques, and on the search for possible correlations between ESI-MS data and other known properties for these compounds. Both the parent drug (PQ, 1) and other PQ imidazolidin-4-ones with additional structural changes, such as acetylation of secondary amino groups (9, 10) or modification of the quinoline ring (10) were included in the study for an assessment of their effect on compound’s properties.. Experimental. Synthesis of the imidazolidin-4-one derivatives. The synthesis and spectral characterization of compounds 4–10 have been reported elsewhere.14,20,21,42 PQ derivatives 4g and 5e, as well as imidazolidin-4-one 11, were successfully prepared by similar methods, respectively, from PQ and from 2-tert-butyl-primaquine (BPQ), the latter prepared as previously described in the literature.43,44. Instrumentation and analytical conditions ESI-MS studies on all compounds were achieved using a Finnigan Surveyor LCQ DECA XP MAX quadrupole ion trap mass spectrometer (San José, CA, USA), utilizing ESI. In the ESI source, nitrogen sheath gas flow was maintained at 20 psi, the capillary temperature was set to 275ºC and the spray voltage was set to 5 kV. Capillary voltage was of 15 V and the tube lens voltage was of 30 V. The instrument was calibrated with caffeine (Aldrich), MRFA (tetrapeptide, Thermo Finnigan), Ultramark 1621 (Lancaster) in the mass range of 195–1821. To induce sample fragmentation, two processes were used: in-source collision-induced dissociation (CID) in the nozzleskimmer region and tandem mass spectrometry (MS/MS). For full details see references.21,22 Briefly, in the first process, fragmentation is induced by colliding sample ions with the background gas in the intermediate-pressure region of the ESI interface. This region is also called “nozzle-skimmer” and pressure gas is about 10–1 mbar. Increasing the voltage between the nozzle and the skimmer (Vs CID) increases the kinetic energy of the ions passing through this region. This raises the energy of collision between the ions and background gas, eventually causing fragmentation.45 MS/MS occurs at the high vacuum region of the mass analyzer; the ion of interest is isolated within the ion trap by ejecting all other ions out of the trap. Then, this ion is accelerated, by applying a high-frequency AC voltage, and collides with a collision gas. The fragmentation ions generated are then detected by a mass scan.45 The collision energy needed to achieve optimum fragmentation efficiency has been shown to follow a linear correlation with m/z. The normalized collision energy (NCE) principle automatically compensates for this mass dependency.46 NCE levels of 10%, 20%, 30% and 40% were tested to establish the optimal NCE value that would allow, through MS2 spectra, analyzing the structural stability of the sample, as this parameter has been used to predict drug metabolites at given NCE values as recommended by the MS manufacturer (25% or 30%).46,47 All spectra were only obtained in positive ionization mode, as compounds under study (basic nitrogen-containing molecules) are barely detectable in the negative ionization mode. Data were collected and analyzed by using the Xcalibur software developed by ThermoFinnigan.. Sample preparation and injection Degassed methanolic solutions of the compounds, containing 0.1% acetic acid, were infused into the ESI probe at a rate.
(3) PQ-derived imidazolidin-4-ones (4-8) by ESI-MS fragmentation techniques, and on the search for possible correlations between ESI-MS data and other known properties for these compounds. Both the parent drug (PQ, 1) andetother PQJ.imidazolidin-4-ones with additional N. Vale al., Eur. Mass Spectrom. 15, 627–640 (2009) structural changes, such as acetylation of secondary amino 629 groups (9, 10) or modification of the quinoline ring (10) were included in the study for an assessment of their effect on compound’s properties.. O. O N. N HN. O. HN. NH2. N H. 1. N NH2. O. O. O. N H. 2. O. 3. HN. N. O. HN. N H. N. NH. O O. R. N. R. O. HN. N. N H. R1. R. 2. 6 6a, R1=H, R2=H 6b, R1=H, R2=CH3 6c, R1=CH3, R2=H 6d, R1=CH3, R2=CH3 6e, R1=CH3, R2=(CH2)2SCH3 6f, R1=H, R2=CH2Ph 6g, R1=H, R2=CH(CH3)2 6h, R1=H, R2=CH2CH(CH3)2. O. N. N. N N. O N. O. N N. HN. N. O. 9. O. NH2 R2 8 8a, R1=H, R2=H 1 2 8b, R =H, R =CH3 8c, R1=H, R2=CH(CH3)2 8d, R1=H, R2=CH2CH(CH3)2 8e, R1=H, R2=CH(CH3)CH2CH3 8f, R1=H, R2=(CH2)2SCH3 8g, R1=H, R2=CH2Ph 8h, R1=CH3, R2=H 8i, R1=CH2CH(CH3)2, R2=H. 7 7a, n=2, R1=H, R2=CH2Ph 7b, n=2, R1=H, R2=CH3 7c, n=2, R1=CH3, R2=H 7d, n=2, R1=CH3, R2=CH3 7e, n=2, R1=CH3, R2=(CH2)2SCH3 7f, n=2, R1=CH(CH3)2, R2=H 7g, n=2, R1=CH2Ph, R2=H 7h, n=2(4-Me), R1=H, R2=CH2Ph 7i, n=3, R1=H, R2=CH2Ph. O. R1. N. 1. O. HN. O. N HN. NH. n. O. n 5 5a, n=1, R1=CH3 5b, n=1, R1=CH(CH3)2 5c, n=1, R1=CH2Ph 5d, n=2, R1=H 5e, n=2, R1=CH3 5f, n=2, R1=CH(CH3)2 5g, n=2, R1=CH2CH(CH3)2 5h, n=2, R1=CH(CH3)CH2CH3 5i, n=2(4-Me), R1=H 5j, n=2(4-Me), R1=CH3 5k, n=2(4-Me), R1=CH(CH3)2 5l, n=3, R1=CH3 5m, n=3, R1=CH(CH3)2 5n, n=3, R1=CH2CH(CH3)2 5o, n=3, R1=CH(CH3)CH2CH3. 4 4a, R1=H 4b, R1=CH3 4c, R1=CH(CH3)2 4d, R1=CH2Ph 4e, R1=CH2CH(CH3)2 4f, R1=CH(CH3)CH2CH3 4g, R1=(CH2)2SCH3. 2. NH. R1. N. NH. N. O O. O. N HN. R1. N. NH2. O. O. N. O. HN. N. 10. of 3 µL min–1. Inclusion of methanol to prepare samples was necessary to obtain good electrospray behavior.48 For those derivatives bearing the amino acid methionine (4g, 6e and 8f), samples were also prepared in degassed acetonitrile and re-analyzed, to check the identity of methanol adducts detected in the previous analyses (cf. the section on In-source CID spectral analyses).. Results and discussion In-source CID spectral analysis. Previous comprehensive ESI-MS fragmentation studies on compounds 4 have revealed that acetone-derived imidazolidin-4-ones, especially smaller ones (R1 = H, Me), are clearly more “fragmentable” in the NSR than those derived from cyclic ketones.21 This has now been confirmed with compounds 4g (PQMetAcetone, R1 = (CH2)2SMe) and 5e. O. C(CH3)3. O. N. R1 N H. 11 11a, R1=H 11b, R1=CH(CH3)2. (PQAlaC6, R1 = Me), not included in that previous work. While at high voltages one could observe��������������������� two major fragmentations of 4g leading to peaks at m/z 175 (6-methoxy-8-aminoquinoline, MAQ) and m/z 132 (7,8-dihidroquinoline, DHQ), as previously reported for other compounds of the same series,21 the in-source CID spectrum of compound 5e exclusively exhibited peaks from species [M + Na]+ and [2M + Na]+. The same general behavior in the NSR was found for the remaining series of PQ-derived imidazolidin-4-ones, that is, for compounds 6–8, as predominant species on the in-source CID spectra were basically the quasi-molecular ions of the original compound, [M + H]+, its sodium adduct, [M + Na]+, its dimer [2M + H]+, or the sodium adduct of the latter, [2M + Na]+, with major fragmentations corresponding to the release of MAQ (m/z 175) or DHQ (m/z 132).22 An interesting observation concerning the behavior of compounds 4–8 in the NSR was the parallelism found between compound resistance to collision-induced fragmentation and.
(4) 630. ESI-MS Characterization of Novel Primaquine Peptidomimetic Derivatives. trend. This strongly suggests that in-source CID spectra might work as a predictive tool for compound reactivity towards hydrolysis under physiological conditions. pgbd4501MeOH #50 RT: 1.36 AV: 1 NL: 1.21E7 T: + p ESI sid=100.00 Full ms [ 50.00-2000.00] 504.93 100. pgbd0315MeOH #50 RT: 1.36 AV: 1 NL: 1.73E6 T: + p ESI sid=80.00 Full ms [ 50.00-2000.00] 160.73 100. [M+H]+. 90. 90. 80. 80 70 Relative Abundance. Relative Abundance. 70. [M+H]+. 60 50 40. 915.67. 30. 469.73. 20 10 0. 502.07. 273.60. 200. 768.27 400. 600. 800. 50. 20. 960.07 1173.53 1000 m/z. 1029.33. 40 30. 825.80. 448.07. 60. 1200. 1334.67 1485.53 1400. 1600. 10. 526.67. 243.67 413.67. 1818.87 1800. 175.67. 2000. 0. 200. 400. 973.53. 658.67 600. 800. 1105.20. 1000 m/z. 1200. 1335.33 1511.60 1400. 1600. 1830.87 1800. 2000. Figure 1. In-source CID spectra at 100 V of 4d (left) and 8g (right). Compound 4d has t1/2 = 31 days in isotonic buffer at pH 7.4 and 37°C, 15,16,19 whereas 8g does not sufferCID any detectable identical Figure 1. In-source spectra atdegradation 100 V of under 4d (left) and conditions. 8g (right). Compound 4d has t =31 days in isotonic 1/2. buffer at pH 7.4 and 37 ºC, whereas 8g does not suffer any detectable degradation under identical conditions.15,16,19. their stability to hydrolysis at physiological pH and T. For Another relevant aspect about the physicochemical properinstance, if we take the in-source CID spectra of compounds ties of the title compounds, which could be traced by ESI-MS 4d (PQPheAcetone, R1 = Bzl) and 8g (PQGlyAcetonePhe, R1=H analysis in the NSR, concerned the presence or absence of We could also detect the minor formation, in the NSR, of dimeric structures derived from and R2 = Bzl) at 100 V (Figure 1), we can see that 4d is clearly ionizable (proton-donating) amino groups in the analyzed less stable than 8g in the NSR. Now, taking their behavior in structures. Ionizable groups/hydrogen-bonding pairs are compounds 4, 5 andpH8, this welast to roles the inbonding of two identicalreacisotonic buffer at physiological andin T,15,16,19 findcase exactlycorresponding known to play key many chemical and enzymatic the same trend. This strongly suggests that in-source CID tions. In the case of PQ-derived imidazolidin-4-ones, we set out 21,22or both secondary amino spectra might workbridged as a predictive tool for compound the [M+H] effect of +blocking monomers by a methylene groupreac(m/z to= analyze 2 × m/z + 12).one These findings tivity towards hydrolysis under physiological conditions. groups in structures 4. This was done by acetylation of one We could also detect the minor formation, in the NSR, of or both the relevant secondary amines in 4a (PQGlyAcetone, arestructures strikingly infrom line with reports McChesney co-workers, who have identified dimeric derived compounds 4, 5 and 8,from in this last R1 = H) to,and respectively, yield structures 9 and 10. N-acetylation case corresponding to the bonding of two identical monomers led to an increase in compound stability towards collision+ minor dimeric metabolites PQ[M + H] produced by Streptomyces rimosus tropicalis, bridged by a methylene group (m/z = 2 ×ofm/z + 12).21,22 induced dissociation in theand NSR,Candida as depicted for compound These findings are strikingly in line with reports from McChesney 10 in Figure 3, where only the sodium adduct of the quasi+ and co-workers, who have identified dimeric metabolites ion of the original , or its dimer, where two molecules of minor N-acetyl-PQ are boundmolecular together through either species, a direct[M + Na] biphenyl-like + of PQ produced by Streptomyces rimosus and Candida tropicalis, [2M + Na] , were seen as predominant species at voltages from where two molecules of N-acetyl-PQ are bound together through 10 V to 100 V. between both C-5 carbons or a methylene brigde between those same eitherbridge a direct biphenyl-like bridgequinoline’s between both quinoline C–5 MS/MS analysis carbons or a methylene brigde between those same quino49,50 49,50 linic carbons. bearing the amino General pathways and (4g application quinolinicInterestingly, carbons. compounds Interestingly, compounds bearingfragmentation the amino acid methionine and acid methionine (4g and 7e) presented in-source CID spectra to isomer identification with an additional peak at m/z = m/z [M + Na]+ + 32 [(Figure 2(a)] MS/MS analysis was tested at four different NCE, which 7e) presented in-source CID spectra with an additional peak at m/z = m/z [M+Na]+ +detected 32 when the samples were prepared in methanol. Ascribing such that all imidazolidin-4-ones underwent visible fragmentation peaks to methanol adducts is the most obvious interpretation. only at NCE ≥ 30%, corroborating the high stability observed in (Figure 2 A) were prepared methanol. Ascribing peaks methanol Adduct formation mustwhen involvethe thesamples Met sulphur atom, as iden- inthe NSR (see the section onsuch In-source CIDto spectral analysis)). tical adducts were not observed for any compounds where Met Moreover, all compounds studied, with the exception of 8 and was absent. In fact, oxidative formation of covalent Met adductsAdduct 10, had loss of MAQ21,22 as the most relevant fragmentation, adducts is the most obvious interpretation. formation must involve the Met sulphur as 51 with aromatic moieties, for example, catechols 3,5-dibromo- the base-peak of all MS2 spectra was invariably observed at 4-nitrosobenzenesulphonic acid52 have been reported. An alter- m/z = m/z [M + H]+–174. atom, as identical adducts were not observed forInany compounds where Met was absent. In native explanation for the peak at m/z = m/z [M + Na]+ + 32 would the case of MS 2 spectra of 8 at NCE = 30%, the basebe Met oxidation in the course of the in-source CID experi- peak was invariably found at m/z=300, independently of 51 fact, oxidative adducts withstructure aromaticof moieties, e.g.,i.e. catechols ments, as oxidation of theformation Met thioetherof to acovalent sulfone is aMet current the exact the compound, of the identity phenomenon in vivo,53–56 which is also chemically or photo- of substituent groups R 1 and R 2. This was a very imporchemically inducible.57 finding, been as it constitutes a major between 3,5-dibromo-4-nitrosobenzenesulphonic acid52tanthave reported. An difference alternative To check which hypothesis was the correct one, new samples compounds 8 and their isomers 6, showing that ESI-MS 2 were prepared in acetonitrile and their spectra obtained, + analysis of these kinds of structure may become a useful explanation for the peak at m/z = m/z [M+Na] + 32 would be Met oxidation in the course of which allowed observing the disappearance of the m/z = m/z tool for isomer identification. The rationale for the different [M + Na]+ + 32 peak [Figure 2(b)], providing the proof that this behavior of compounds 6 vs 8 was based on the disruption the CIDofexperiments, as oxidation ofof the thioether toringa of sulfone is the a current was in factin-source due to formation methanol adducts. the iMet midazolidin-4-one 8 to yield iminium ion.
(5) N. Vale et al., Eur. J. Mass Spectrom. 15, 627–640 (2009). 631. Figure 2. In-source CID spectra of 7e (PQAlaMetC6, MW 541) at 25 V in (a) methanol and (b) acetonitrile.. 12 (Scheme 1) that readily undergoes a cyclization-elimination reaction to produce the detected propyliminium ion 13 and a neutral diketopiperazine (DKP, 14). This fragmentation pathway is similar to that described as the “diketopiperazine pathway” for MS/MS backbone fragmentations of oligopeptides58–66 and, as mentioned before, can be regarded as a valuable instrument for unequivocal distinction between. terminal (6, PQXaaPro mimetics) and “internal” (8, PQProXaa mimetics) imidazolidin-4-one isomers.22. Levels of unfragmented original species and application to stability predictions Another analyzed parameter was the relative abundance of the original (unfragmented) species still detected on MS2 spectra.
(6) 632. ESI-MS Characterization of Novel Primaquine Peptidomimetic Derivatives. pgbd0420MeOH #26 RT: 0,68 AV: 1 NL: 7,69E7 T: + p ESI sid=10,00 Full ms [ 50,00-2000,00] 463,93 100 95. [M+Na]+. 90 85 80 75 70. Relative Abundance. 65 60 55 50 45 40 35 30 25 20 15. 903,27. 10 5 0. 175,60 285,93 348,40 200. 539,60. 400. 600. 680,67. 853,60. 979,40. 800. 1176,27. 1000 m/z. 1323,40 1462,00 1593,00 1716,33. 1200. 1400. 1600. 1800. 2000. pgbd0420MeOH #73 RT: 2,01 AV: 1 NL: 2,19E7 T: + p ESI sid=50,00 Full ms [ 50,00-2000,00] 463,93 100 95 90 85 80 75 70. Relative Abundance. 65 60 55 50 45 40 35. 903,47. 30. [2M+Na]+. 25 20 15. 348,73. 10 5. 286,00. 539,67. 175,67. 0. 624,67 724,27 860,73. 200. 400. 600. 920,60. 800. pgbd0420MeOH #86 RT: 2,36 AV: 1 NL: 1,99E6 T: + p ESI sid=100,00 Full ms [ 50,00-2000,00]. 1147,87 1232,13. 1000 m/z. 1200. 1413,53 1547,73 1634,60 1400. 1600. 1849,67 1933,00 1800. 2000. 903,67. 100 95 90 463,87. 85 80 75 70. Relative Abundance. 65 60 55 50 45 40 35 30. 175,60. 25 20 15. 285,80 132,47. 10. 349,07. 5 0. 200. 400. 916,60. 479,47 539,67. 600. 763,00. 800. 944,60. 1000 m/z. 1202,73. 1200. 1300,80. 1485,07. 1400. 1624,80 1600. 1860,60 1800. 2000. Figure 3. In-source CID spectra for 10 (MW 440) on the NSR at 10 V (top), 50 V (middle) and 100 V (bottom).. Figure 3. In-source CID spectra for 10 (MW 440) on the NSR at 10 (top), 50 (middle) and 100 V (bottom)..
(7) N. Vale et al., Eur. J. Mass Spectrom. 15, 627–640 (2009). 633. O. O R O. N HN. N R1. N. O. HN R. N. NH2. N. N H. 2. R1 NH3. O. 8. 2. O. 6. O. O. O R1. N. O. HN. N. N H N R1 H 2N. O. +. HN. NH HN. N H. R2 O. R2. 12. 13. 14. m/ z 300. Scheme 1. Fragmentation pathway of 8 leading to formation of its major MS2-fragment, detected at m/z 300. 2. Scheme 1. Fragmentation pathway of 8 leading to formation of its major MS -fragment, detected at m/z 300.. at NCE = 30% b) forlevels thoseofcompounds sharing identical major Correlation between imidazolidin-4-one ring unfragmented original species and application to stability predictions fragmentation phenomena (loss of MAQ or 2-tBu-MAQ). As stability in MS/MS and useful physicochemical shown in TableAnother 1, both the parent drug, 1, and was its 2-tert-butyl analysed parameter the relative descriptors abundance of the original (unfragmented) derivative, BPQ, were quantitatively fragmented under these In our most recent work on compounds 8 as PQProXaa conditions (Figure 4), therefore being the of the mimetics, we came across a striking species still detected onleast MS2stable spectra at set. NCE=30% for those compounds sharing observation identical concerning On the other hand, their imidazolidin-4-one derivatives followed the fact that the relative abundance of an important 2 t the stability order 11 > 4 > 6. This ranking agrees with ouroffind-fragment wasAs inversely to the size of the major fragmentation phenomena (loss MAQ MS or 2Bu-MAQ). shown proportional in Table 1, both ings on the relative stability of compounds 4 and 6 towards side chain in the variable amino acid Xaa.22 This was reflected hydrolysis at physiological pH and T,15,16,19,20 again suggesting a perfectly linear correlation (y = – 0.66n + 2.33; r2 = 0.997, the parent drug, 1, and its 2-tert-butyl derivative,byBPQ, were quantitatively fragmented under that ESI-MS fragmentation behavioral patterns might work as a n = 5) between the logarithm of the fragment’s relative abunpredictor tool for compound stability in aqueous media. being the least dance (y) and the set. Charton parameter, n,67their associated to the these conditions (Figure 4), therefore stable of the On the other hand, 22 Interestingly, while insertion of the 2-tert-butyl substituent side chain of each different Xaa. These findings prompted in PQ did not seem to exert any stabilizing effect, the opposite us to order engage into an This empirical search for with other correlations imidazolidin-4-one derivatives followed the stability 11>4>6. ranking agrees was found when considering the corresponding imidazolidin- between MS2-fragmentation behavior of the other two families 4-one derivatives and 4a. Although the physico–chemical of PQ imidazolidin-4-ones, namely, PQPro mimetics (repreour 11findings on the relative stability of compounds 4 and 6 towards hydrolysis at meaning of such a difference is not evident, 11 is clearly more sented by series 4 and 5) and PQXaaPro mimetics (represented stable towardsphysiological fragmentation pH thanand 4a (Figure 5). again suggesting by series and 7) with relevant physico–chemical that 6ESI-MS fragmentation behavioural descriptors. T,15,16,19,20 Compounds 8 had a markedly different major fragmentation Results obtained are described below. pathway and, therefore, were excluded this evaluation. In patterns might work as from a predictor tool for compound stability in aqueous media. the case of the acetyl derivatives 9 and 10, these were practi- Imidazolidin-4-one ring opening in PQPro mimetics cally intact atInterestingly, NCE 30% (relative the 2-tert-butyl original In order to establish parallel ring whileabundance insertion ofof the substituent in PQ dida not seemfortoimidazolidin-4-one exert any species≈100%), reinforcing that N-acetylation produces highly opening in aqueous media,16 we set out to explore the extent of 2 stable molecules. -induced ring opening on a series of compounds 5, taken stabilizing effect, the opposite was found when MS considering the corresponding imidazolidin-. 4-one derivatives 11 and 4a. Though the physicochemical meaning of such difference is not Table 1. Relative abundance of the original species at NCE = 30% on MS2.. evident, 11 is clearly more stable towards fragmentation than 4a (Figure 5). Compound. 2-tBu-primaquine 1. Abbreviation. % initial compound after MS2 fragmentation. BPQ. 0. PQ. 0. 6a. PQGlyGlyAcetone. 10.40. 4a. PQGlyAcetone. 23.86. 11a. BPQGlyAcetone. 71.27.
(8) 1 PQ 0 6a PQGlyGlyAcetone 10.40 4a PQGlyAcetone 23.86 imidazolidin-4-one derivatives followed the stability order 11>4>6. This ranking agrees with our findings on the 11a BPQGlyAcetone 71.27 relative stability of compounds 4 and 6 towards hydrolysis at physiological pH and T,15,16,19,20 again suggesting that 634 ESI-MS Characterization of Novel Primaquine Peptidomimetic Derivatives ESI-MS fragmentation behavioural patterns might work as a predictor tool for compound stability in aqueous media. Interestingly, while insertion of the 2-tert-butyl substituent in PQ did not seem to exert any stabilizing effect, the opposite was found when considering the corresponding imidazolidin-4-one derivatives 11 and 4a. Though the pgbd0103MeOH #85 RT: 2,15 AV: 1 NL: 2,95E7 T: + p ESI Full ms2 260,00@30,00 [ 70,00-2000,00] physicochemical meaning 243,17 of such difference is not evident, 11 is clearly more stable towards fragmentation than 100 4a (Figure 5). 90 Table 1. Relative abundance of the original species at NCE=30% on MS2. 80 pgbd1409MeOH_071003113750 #146 RT: 2.14 AV: 1 NL: 3.10E6 T: + p ESI Full ms2 316.00@30.00 [ 85.00-500.00] 299.29 100 O. O. 90. O. O. 231.36. 80. N. HN. 40 30. m/z 85. 0. Abbreviation. m/z 298. 60. NH2. 20 10. Compound. m/z 242. Relative Abundance. Relative Abundance. NH2. 50. m/z 230. % initial compound after MS2 fragmentation 50 m/z 174 BPQ 0 2-tBu-primaquine 40 175,38 1 PQ 0 30 imidazolidin-4-one derivatives followed the stability order 11>4>6. This ranking agrees with our findings on the 20 6a PQGlyGlyAcetone 10.40 relative stability of compounds 4 and 6 towards hydrolysis at physiological pH and T,15,16,19,20 again suggesting that 10 4a PQGlyAcetone 23.86 ESI-MS fragmentation behavioural patterns might work as a predictor tool for compound stability in aqueous 315.12 215,43 226.90 243.20 0 11a BPQGlyAcetone 71.27 media. 150 200 250 300 350 400 450 500 100 150 200 250 300 350 N. 60. HN. C(CH3)3. NH2. 70. 70. C(CH3)3. N. N. 86,17 100. 400. m/z m/z Interestingly, while insertion of the 2-tert-butyl substituent in PQ did not seem to exert any stabilizing effect, the 2 opposite was found when considering the corresponding imidazolidin-4-one derivatives 11 and 4a. Though the Figure MSMS spectra for PQ (left) BPQ (right) at NCE = 30%. for PQand (left) and BPQ (right) at NCE=30%. Figure4. 4. 22 spectra of such difference not evident, 11 is clearly more stable towards fragmentation than Figure 4. MSphysicochemical spectra formeaning PQ (left) and BPQ is(right) at NCE=30%. 4a (Figure 5). Table 1. Relative abundance of the original species at NCE=30% on MS2.. Relative Abundance. 90 pgbd0314MeOH #79 RT: 2.11 AV: 1 NL: 5.09E6 T: + p ESI Full ms2 357.00@30.00 [ 95.00-2000.00] 183.15 80 m/z 182 100 N 70 90. 80. Relative Abundance. 70 60 50 40. 60. m/z 182. O. Compound. % initial compound after MS fragmentation BPQ 0 N 90 pgbd1704MeOH #27-32 RT: 0.63-0.73 AV: 6 NL: 5.34E6 PQ 0 T: + p ESI Full ms2 413.00@30.00 [ 110.00-2000.00] NH O 183.33 80 m/z 182 PQGlyGlyAcetone 100 10.40 O 70 PQGlyAcetone 23.86 N 90 BPQGlyAcetone 71.27 60 NH 231.40 Abbreviation. 2-tBu-primaquine 1 6a 4a NH11a O NH. 70 Figure 4. MS2 spectra for PQ (left) and BPQ (right) at NCE=30%.. 40. 40 60. 20. m/z 182. 80. 50. 30. 500. pgbd1704MeOH #27-32 RT: 0.63-0.73 AV: 6 NL: 5.34E6 T: + p ESI Full ms2 413.00@30.00 [ 110.00-2000.00] 2 O 183.33 100. Relative Abundance Relative Abundance. pgbd0314MeOH #79 RT: 2.11 AV: 1 NL: 5.09E6 T: + p ESI Full ms2 357.00@30.00 [ 95.00-2000.00] 183.15 100 N. 450. N. O. 50. NH2. m/z 230 231.40. + m/z2 [PQ+H] m/zcompounds M-NH3 Figure 5. MS spectra for 4a (left) and 11a (right) at 30 NCE=30%.. N. 414.27. 414.27. m/z [M+H]+. m/z [M+H]+. C(CH3)3. m/z M-NH3 m/z [BPQ+H]+ C(CH3)3. 395.40. m/z M-NH3 20 m/z 230 m/z [BPQ+H]+ 299.47 40 Compounds 8260.12 had a markedly different major fragmentation pathway and, therefore, were excluded from this 357.19. m/z. [M+H]+. 339.22. 50. NH2. 316.33. 243.22 381.53395.40 285.47 10 + evaluation. the case of325.12 the acetyl derivatives 9 and 10, these30 were practically m/z In [PQ+H] m/z M-NH 197.33 intact at NCE 30% (relative 229.32 3 342.33 286.20 271.60 143.33 426.27 316.33 357.19 abundance of the original species≈100%), reinforcing that N-acetylation produces highly stable molecules. m/z [M+H]+ 0 0 100 150 200 250 300 350 400 450 500 20 150 200 250 300 350 400 450 500 20 Correlation 260.12 between imidazolidin-4-one ring stability in tandem-MS and useful physicochemical descriptors m/z m/z 299.47 339.22 243.22 285.47 we came across a striking observation 381.53 10 10 175.43 In our most recent work on compounds 8 as PQProXaa mimetics, 197.33 325.12 229.32 2-fragment was inversely Figure MS2 spectra forthe compounds 4arelative (left) and 11a (right) atimportant NCE = 30%. 342.33to the 127.07 5. 161.73 286.20 271.60 proportional concerning fact that the abundance of an MS 143.33 426.27 0 0 22 This 2of the side 150 250 300 350 450 500 100 200 250 chain 300 350 400 500was reflected size in the variable amino acid 450 Xaa. by 200 a perfectly linear correlation (y = – 400 spectra for compounds 4a (left) and 11a (right) at NCE=30%. Figure1505. MS m/z m/z 0.66ν + 2.33; r2=0.997, n=5) between the logarithm of the fragment’s relative abundance (y) and the Charton parameter, ν,67 associated to the side chain of each different Xaa.22 These findings prompted us to engage into an empirical search for other correlations between MS2-fragmentation behaviour of the other two families of PQ 2 Figure 5. MSimidazolidin-4-ones, spectra compounds 4a (left) and 11a (right) NCE=30%. as representative of PQProfor mimetics. series was(represented chosen peptides and amino acid derivatives 68–70 namely,This PQPro mimetics byfragmentations series at 4 and 5) and of PQXaaPro mimetics (represented series 6 and 7) with descriptors.those Resultsbearing obtained are described below. 71 Indeed, every to include different aminobyacids whose siderelevant chainsphysicochemical (R1) are including Xaa-Pro moieties. Imidazolidin-4-one in PQPro associated to distinct values ofring theopening Charton stericmimetics parameter, MS2 spectrum of the compound series analyzed displayed In order to establish a parallel for imidazolidin-4-one ring opening in aqueous media,16 we set out to explore the n. Previous studies have shown that protonated PQ imidazoa peak compatible with structure 15, which reinforced our extent of MS2-induced ring opening on a series of compounds 5, taken as representative of PQPro mimetics. This 1 lidin-4-ones undergo ring opening in aqueous buffers at 60ºC assumption. Consequently, we considered that the abundance series was chosen to include different amino acids whose side chains (R ) are associated to distinct values of the Charton parameter, Previous studies have shown imidazolidin-4-ones undergorelative ring in a fashion similar to steric the first step onν. Scheme 2,16 which was thatofprotonated 15 couldPQreflect the compound’s stability and be opening in aqueous buffers at 60 ºC in a fashion similar to the first step on Scheme 2,16 which was recently recently confirmed for compounds 6 and 197.19 Therefore, we used to find any correlation between compound stability and confirmed for compounds 6 and 7. Therefore, we hypothesized that such ring opening could easily occur in the hypothesized that such ring opening could easily occur in the s course of MS2-fragmentations, being followed by ammonia losstructure. to yield ion 15. This hypothesis was based on the fact that ammonia loss is a common phenomenon on MSn-induced of peptidesabundance and amino acid course of MS2-fragmentations, being followed by ammonia Thefragmentations log of the relative of 15 was represented 68-70 including those bearing Xaa-Pro moieties. 71 Indeed, every MS2 spectrum of the compound series 1 loss to yield ionderivatives 15. This hypothesis was based on the fact that against the value of n associated to each R (Table 2), resulting analyzed displayed a peak compatible with structure 15, which reinforced our assumption. Consequently, we ammonia loss considered is a common phenomenon on MSn-induced in the perfectly linear correlation displayed on Figure 6. that the abundance of 15 could reflect the compound’s relative stability and be used to find any correlation between compound stability and structure. 10. 30. 175.43. 127.07 161.73. Compounds 8 had a markedly different major fragmentation pathway and, therefore, were. excluded from thisa evaluation. In the case of fragmentation the acetyl derivatives and 10, these were Compounds 8 had markedly different major pathway9 and, therefore, were practically intact NCE 30% (relative abundance the original species≈100%), excluded from thisat evaluation. In the case of theofacetyl derivatives 9 and 10, reinforcing these were that N-acetylation produces highly stable molecules.. O. O. O R1 N. H NH 2. R1 N. N. H. O R1. N. NH2 NH 3. 5. 15. Scheme 2. Imidazolidin-4-one ring opening after protonation of its secondary amine, to yield ion 15.. Scheme 2. Imidazolidin-4-one ring opening after protonation of its secondary amine, to yield ion 15.. R1.
(9) N. Vale et al., Eur. J. Mass Spectrom. 15, 627–640 (2009). 635. Table 2. Relative abundance of species 15 (MH+ – 17) resulting from MS2 fragmentation of compounds 5d, 5e, 5f and 5h at NCE = 30% and the Charton steric parameter, n,67 associated to each R1.. R1. Compound. Abbreviation. 5d. PQGlyC6. H. 5e. PQAlaC6. CH3. 5f. PQValC6. CH(CH3)2. 5h. PQIleC6. CH(CH3)CH2CH3. Charton steric parameter for R1, ν. Relative abundance (%) of 15. log (relative abundance). 0. 33.36. 1.52. 0.52. 16.08. 1.21. 0.76. 10.67. 1.03. 1.02. 8.68. 0.94. 5e 5f 5h. have been found between compound reactivity in solution under mild conditions (aqueous media at physiological pH and T) and compound fragmentation behavior in the course of MS/MS experiments based on ESI techniques. These findings open a whole new perspective on the application of ESI-MS techniques to the characterization of drugs bearing PQAlaC6 CH3 0.52structural motif. 16.08 1.21 an imidazolidin-4-one or similar PQValC6 PQIleC6. CH(CH3)2 CH(CH3)CH2CH3. 0.76 1.02. 10.67 8.68. 1.03 0.94. Imidazolidin-4-one ring opening in PQXaaPro mimetics. Figure 6. Plot of log(relative abundance of 15) versus the Charton steric parameter, ν, associated to the amino by thecompounds above results, we searched for similar correacid’s side Motivated chain R1 of the original 5.. lations in PQXaaPro mimetics, taking compounds 6 as repre-. The negative slope obtained indicates that steric crowding imposed by the amino acid’s side chain R 1 unfavors or sentative of15.this To that purpose, we selected mostmedium, as delays the formation of ion This family. is astonishingly coincidental with our previous findingsthe in aqueous reported in reference 16. In this former work, a parallel correlation was found, for the same compounds, between relevant fragment reflecting imidazolidin-4-one ring disruplog kneut (apparent first-order rate constant for ring opening in the neutral imidazolidin-4-ones 5) and the Charton steric parameter r2=0.993, n=4).16 This is a relevant finding,value as there75 is noamu other example neut= – 1.30ν – 0.75; 6, tion (log on kcompounds associated with an m/z in the literature where parallel quantitative structure-reactivity correlations have been found between compound lower than that of the corresponding unfragmented quasireactivity in solution under mild conditions (aqueous media at physiological pH and T) and compound Figure 6. Plot of log(relative abundance of 15) versus the + 22 fragmentation behaviour in ion the course of tandem-MS experiments based on ESI techniques. These findings open a molecular [M + H] . Such mass loss corresponds to ring Charton steric parameter, n, associated to the amino acid’s whole new perspective on the application of ESI-MS techniques to the characterization of drugs bearing a opening with the departure of isopropylamine plus dehydraimidazolidin-4-one or similar structural motif. side chain R1 of the original compounds 5. Imidazolidin-4-one ring opening in PQXaaPro mimetics tion, to yield ion 16, so we took the relative abundance of this Motivated by the above results, we searched for similar correlations in PQXaaPro mimetics, taking compounds 6 ion as an family. indirect measure the relative ring stabilities within as representative of this To that purpose, weof selected the most relevant fragment reflecting imidazolidin4-one ring disruption on compounds 6, associated to an m/z value 75 a.m.u. lower than that of the corresponding a subset of compounds 6 having Gly directly attached to PQ unfragmented quasi-molecular ion [M+H]+.22 Such mass loss corresponds to ring opening with departure of isopropylamine plus dehydration, to yield ion 16, so weacids took the forming relative abundance this ion as an indirect The negative slope obtained indicates that steric crowding (R1 = H) and varying amino the ofimidazolidinmeasure of the relative ring stabilities within a subset of compounds 6 having Gly directly attached to PQ (R1=H) 2 imposed by the amino acid’s side chain R1 does not favor,andorvarying4-one ring (R = H, Me, Bzl, iPr and iBu). amino acids forming the imidazolidin-4-one ring (R 2=H, Me, Bzl, iPr and iBu).. delays, the formation of ion 15. This is astonishingly coinO cidental with our previous findings in aqueous medium, as reported in Reference 16. In this former work, a parallel N O H HN N correlation was found, for the same compounds, between R N H log kneut (apparent first-order rate constant for ring opening 16 in the neutral imidazolidin-4-ones 5) and the Charton steric parameter (log kneut = – 1.30n – 0.75; r2 = 0.993, n = 4).16 This is a The correlation between the relative abundance of ion 16, for relevant finding, as there is no other example in the literature the subset mentioned above, and the value of ν associated to 2 The correlation the relative(Table abundance of ion 16, for abundance the subset mentioned where parallel quantitative structure–reactivity correlations each Rbetween , was analyzed 3). The relative of 16 above, 2. and the value of ν associated to each R2, was analyzed (Table 3). The relative abundance of . The linear fitand is very good if all the five 16 was again of inversely proportional R2at Table 3. Relative abundance of species 16 resulting from MS2 fragmentation compounds 6a, 6b,to6f,the 6gsize andof6h NCE = 30% Charton steric parameters, n,67 associated to each R2. 2 points are considered (r =0.96, n=5) and becomes excellent when one of those points. Compound. Abbreviation. 6.1. PQGlyaa2Acetone. R2. H. 6.2. CH3. 6.6. CH2Ph. 6.7 6.8. Charton steric. Relative abundance. log (relative. 2 1 2=0.99, n=4), reinforcing the significance of the correlation found =iPr) is excluded (Rparameter for R(r ,n (%) of 16 abundance). 0 more, this correlation perfectly 18.68matches our previous1.27 (Figure 7). Once findings concerning 0.52. 13.53. 1.17. 0.70. 12.15. 1.08. CH(CH3)2. 0.76. 10.15. 1.01. CH2CH(CH3)2. 0.98. 9.63. 0.98. the relative rates of ring opening for compounds 6 in aqueous medium.19.
(10) Compound. Abbreviation. 6.1 6.2 6.6 636 6.7 6.8. abundance abundance) (%) of 16 H 0 18.68 1.27 CH3 0.52 13.53 1.17 CH2Ph 0.70 12.15 1.08 ESI-MS Characterization of Novel Primaquine Peptidomimetic Derivatives CH(CH3)2 0.76 10.15 1.01 CH2CH(CH3)2 0.98 9.63 0.98 R. PQGlyaa2Acetone. parameter for R1, ν. 2,50. 2,00. y = -0,29x + 1,28 R2 = 0,99. log rel. abundance ion at m/z M-75 (%). 1,60 1,20. 2,00. 0,80 0,40. 1,50. 1,00. 0,00 0,00. 0,40. 0,80. 1,20. y = -0,30x + 1,28 2. R = 0,96. 0,50. 0,00 0,00. 0,20. 0,40. 0,60. 0,80. 1,00. 1,20. Charton Parameter, ν. Figure 7. Plot of log(relative abundance of 16) versus the Charton steric parameter, n, associated to the amino acid’s side chain R2 of the original compounds 6. The insert shows the plot obtained when 6g is excluded from the correlation analysis.. was again inversely proportional to the size of R2. The linear fit is very good if all the five points are considered (r2 = 0.96, n = 5) and becomes excellent when one of those points (R2 = iPr) is excluded (r2 = 0.99, n = 4), reinforcing the significance of the correlation found (Figure 7). Once more, this correlation perfectly matches our previous findings concerning the relative rates of ring opening for compounds 6 in an aqueous medium.19 Finally, we would like to stress that four to five different compounds were used to establish each one of the above correlations, as the use of four to five experimental points is a common procedure to establish these kinds of structure–[re]activity correlation in therapeutically relevant compounds.72–75. Conclusion In-source CID and MS/MS spectral analyses of peptido mimetic imidazolidin-4-one derivatives of an antimalarial drug, primaquine, were carried out and are described in the present report. In-source CID spectra were found to reflect the overall compound’s relative reactivity under physiological conditions, as compared to data from previous stability studies in aqueous media. Moreover, MS/MS analysis provided a means to distinguish between isomeric PQXaaPro and PQProXaa mimetics, as key fragmentations were different between both families, which only differed at the position where the imidazolidin4-one ring was inserted. Last, but not least, correlations were found between the relative abundances of key MS2-generated fragments and relevant physico–chemical descriptors, namely, the Charton steric parameter associated to amino acid side. chains. To the best of our knowledge, nothing of this kind has been reported by others. Overall, the present work is relevant to consolidate ESI-MS as a key tool for drug analysis and characterization. Specifically in what concerns therapeutically relevant agents bearing the imidazolidin-4-one scaffold or related peptidomimetic structural motifs, our findings show that ESI-MS techniques, both through in-source CID spectra or MS/MS analysis, are reliable tools for stability predictions in aqueous media at physiological pH and T, providing that a suitable choice of key fragmentations is made. As far as we know, this type of correlation analysis has never been approached by other authors.. Acknowledgments Thanks are due to Fundação para a Ciência e a Tecnologia (FCT, Portugal) for financial support through Project PTDC/ QUI/65142/2006. NV thanks FCT for Post-Doctoral fellowship SFRH/BPD/48345/2008. PG and RM thank FCT for support through pluriannual funding to CIQUP and iMed.UL, respectively.. References 1. M. Yamashita and J.B. Fenn, “Negative ion production. with the electrospray ion source”, J. Phys. Chem. 88, 4671 (1984). doi: 10.1021/j150664a046 2. W.F. Smyth, “Electrospray ionisation-mass spectrometry (ESI-MS) and liquid chromatography-electrospray ionisation-mass spectrometry (LC-ESI-MS) of selected.
(11) N. Vale et al., Eur. J. Mass Spectrom. 15, 627–640 (2009). pharmaceuticals”, Curr. Pharm. Anal. 2, 299 (2006). doi: 10.2174/157341206777934644 3. B.L. Tekwani and L.A. Walker, “8-Aminoquinolines: future role as antiprotozoal drugs”, Curr. Opin. Infect. Dis. 19, 623 (2006). doi: 10.1097/QCO.0b013e328010b848 4. L.J.C. Bolchoz, R.A. Budinsky, D.C. Mcmillan and D.J. Jollow, “Primaquine-induced hemolytic anemia: formation and hemotoxicity of the arylhydroxylamine metabolite 6-methoxy-8-hydroxylaminoquinoline”, J. Pharmacol, Exp. Ther. 297, 509 (2001). 5. G.W. Mihaly, S.A. Ward, G. Edwards, M. L’Eorme and A.M. Breckenridge, “Pharmacokinetics of primaquine in man: identification of the carboxylic acid derivative as a major plasma metabolite”, Br. J. Clin. Pharmacol. 17, 441 (1984). 6. L. Constantino, P. Paixão, R. Moreira, M.J. Portela, V.E. do Rosário and J. Iley, “Metabolism of primaquine by liver homogenate fractions. Evidence for MAO and Cyt P450 involvement in the oxidative deamination of primaquine to carboxyprimaquine”, Exp. Toxic. Pathol. 51, 299 (1999). 7. J. Hofsteenge, A. Capuano, R. Altszuler and S. Moore, “Carrier-linked primaquine in the chemotherapy of malaria”, J. Med. Chem. 29, 1765 (1986). doi: 10.1021/ jm00159a034 8. A. Philip, J.A. Kepler, B.H. Johnson and F.I. Carroll, “Peptide derivatives of primaquine as potential antimalarial agents”, J. Med. Chem. 31, 870 (1988). doi: 10.1021/ jm00399a032 9. R. Borissova, B. Lammek, P. Stjärnkvist and I. Sjöholm, “Biodegradable microspheres. 16. Synthesis of primaquine-peptide spacers for lysosomal release from starch microparticles”, J. Pharm. Sci. 84, 249 (1995). doi: 10.1002/jps.2600840226 10. R. Borissova, P. Stjärnkvist, M.O. Karlsson and I. Sjöholm, “Biodegradable microspheres. 17. Lysosomal degradation of primaquine-peptide spacer arm”, J. Pharm. Sci. 84, 256 (1995). doi: 10.1002/jps.2600840227 11. M.C. Chung, M.F. Gonçalves, W. Colli, E.I. Ferreira and M.T.M. Miranda, “Synthesis and in vitro evaluation of potential antichagasic dipeptide prodrugs of primaquine”, J. Pharm. Sci. 86, 1127 (1997). doi: 10.1021/js970006v 12. M.J. Portela, R. Moreira, E. Valente, L. Constantino, J. Iley, J. Pinto, R. Rosa, P. Cravo and V.E. do Rosário, “Dipeptide derivatives of primaquine as transmissionblocking antimalarials: effect of aliphatic side-chain acylation on the gametocytocidal activity and on the formation of carboxyprimaquine in rat liver homogenates”, Pharm. Res. 16, 949 (1999). doi: 10.1023/A:1018922425551 13. S. Romeo, M. Dell’Agli, S. Parapini, L. Rizzi, G. Galli, M. Mondani, A. Sparatore, D. Taramelli and E. Bosisio, ‘Plasmepsin II inhibition and antiplasmodial activity of Primaquine–Statine “double-drugs”’, Bioorg. Med. Chem. Lett. 14, 2931 (2004). doi: 10.1016/j.bmcl.2004.03.030 14. P. Gomes, M.J. Araújo, M. Rodrigues, N. Vale, Z. Azevedo, J. Iley, P. Chambel, J. Morais and R. Moreira, “Synthesis of imidazolidin-4-one and 1H-imidazo[2,1-a]isoindole-. 637. 2,5(3H,9bH)-dione derivatives of primaquine: scope and limitations”, Tetrahedron 60, 5551 (2004). doi: 10.1016/j. tet.2004.04.077 15. M.J. Araújo, J. Bom, R. Capela, C. Casimiro, P. Chambel, P. Gomes, J. Iley, F. Lopes, J. Morais, R. Moreira, E. de Oliveira, V. do Rosário and N. Vale, “Imidazolidin-4one derivatives of primaquine as novel transmissionblocking antimalarials”, J. Med. Chem. 48, 888 (2005). doi: 10.1021/jm0494624 16. P. Chambel, R. Capela, F. Lopes, J. Iley, J. Morais, L. Gouveia, J.R.B. Gomes, P. Gomes and R. Moreira, “Reactivity of imidazolidin-4-one derivatives of primaquine: implications for prodrug design”, Tetrahedron 62, 9883 (2006). doi: 10.1016/j.tet.2006.08.026 17. R. Ferraz, J.R.B. Gomes, E. de Oliveira, R. Moreira and P. Gomes, “Unanticipated stereoselectivity in the reaction of primaquine alpha-aminoamides with substituted benzaldehydes: computational and experimental study”, J. Org. Chem. 72, 4189 (2007). doi: 10.1021/jo0703202 18. N. Vale, M.S. Collins, J. Gut, R. Ferraz, P.J. Rosenthal, M.T. Cushion, R. Moreira and P. Gomes, “AntiPneumocystis carinii and antiplasmodial activities of primaquine-derived imidazolidin-4-ones”, Bioorg. Med. Chem. Lett. 18, 485 (2008). doi: 10.1016/j. bmcl.2007.11.105 19. N. Vale, F. Nogueira, V.E. do Rosário, P. Gomes and R. Moreira, “Primaquine dipeptide derivatives bearing an imidazolidin-4-one moiety at the N-terminus as potential antimalarial prodrugs”, Eur. J. Med. Chem. 44, 2506 (2009). doi: 10.1016/j.ejmech.2009.01.018 20. N. Vale, J. Matos, J. Gut, F. Nogueira, V. do Rosário, P.J. Rosenthal, R. Moreira and P. Gomes, “Imidazolidin-4one peptidomimetic derivatives of primaquine: Synthesis and antimalarial activity”, Bioorg. Med. Chem. Lett. 18, 4150 (2008). doi: 10.1016/j.bmcl.2008.05.076 21. N. Vale, R. Moreira and P. Gomes, “Characterization of primaquine imidazolidin-4-ones with antimalarial activity by electrospray ionization-ion trap mass spectrometry”, Int. J. Mass Spectrom. 270, 81 (2008). doi: 10.1016/j. ijms.2007.12.006 22. N. Vale, J. Matos, R. Moreira and P. Gomes, “Electrospray ionization-ion trap mass spectrometry study of PQAAPro and PQProAA mimetic derivatives of the antimalarial primaquine”, J. Am. Soc. Mass Spectrom. 19, 1476 (2008). doi: 10.1016/j. jasms.2008.06.019 23. S.K. Kokpol, S.V. Hannongbua, N. Thongrit, S. Polman, B.M. Rode and M.G. Schwendinger, “Analysis of structure activity for primaquine antimalarial–drugs by quantum pharmacological approach”, Anal. Sci. 4, 565 (1988). doi: 10.2116/analsci.4.565 24. S. Kristensen, L. Grinberg and H.H. Tønnsen, “Photoreactivity of biologically active compounds: XI. Primaquine and metabolites as radical inducers”, Eur. J. Pharm. Sci. 5, 139 (1997). doi: 10.1016/S09280987(97)00268-6.
(12) 638. ESI-MS Characterization of Novel Primaquine Peptidomimetic Derivatives. 25. V.K. Dua, S.N. Sinha and V.P. Sharma, “Chromatographic. studies of peroxydisulphate oxidation products of primaquine”, J. Chromatogr. B 708, 316 (1998). doi: 10.1016/S0378-4347(97)00639-7 26. S. Kristensen, K. Nord, A.L. Orsteen and H.H. Tønnsen, “Photoreactivity of biologically active compounds, XIV: influence of oxygen on light induced reactions of primaquine”, Pharmazie 53, 98 (1998). doi: 10.2116/ analsci.18.595 27. T. Koide and K. Ueno, “Enantiomeric separations of cationic and neutral compounds by capillary electro chromatography with monolithic chiral stationary phases of b-cyclodextrin-bonded negatively charged polyacrylamide gels”, J. Chromatogr. A 893, 177 (2000). doi: 10.1016/S0021-9673(00)00699-3 28. C. Zhang, C. Zhu, X. Lin, F. Gao and Y. Wei, “Enantiomeric separation of primaquine, an anti-malarial drug, by cyclodextrin-modified micellar electrokinetic capillary chromatography”, Anal. Sci. 18, 595 (2002). doi: 10.2116/ analsci.18.595 29. J. Lal, N. Mehrotra and R.C. Gupta, “Analysis and pharmacokinetics of bulaquine and its major metabolite primaquine in rabbits using an LC-UV method—a pilot study”, J. Pharm. Biomed. Anal. 32, 141 (2003). doi: 10.1016/S0731-7085(03)00033-5 30. A.K. Dwivedi, D. Saxena and S. Singh, “HPLC and HPTLC assays for the antimalarial agents chloroquine, primaquine and bulaquine”, J. Pharm. Biomed. Anal. 33, 851 (2003). doi: 10.1016/S0731-7085(03)00355-8 31. S.N. Sinha and V.K. Dua, “Fast atom bombardment mass spectral analysis of three new oxidative products of primaquine”, Int. J. Mass Spectrom. 232, 151 (2004). doi: 10.1016/j.ijms.2003.11.013 32. I. Brondz, D. Mantzilas, U. Klein, D. Ekeberg, E. Hvattum, M.N. Lebedeva, F.S. Mikhailitsyn, G.D. Souleimanov and J. Røe, “Nature of the main contaminant in the anti malaria drug primaquine diphosphate: a qualitative isomer analysis”, J. Chromatogr. B 800, 211 (2004). doi: 10.1016/j.jchromb.2003.09.042 33. R. Bortocan and P.S. Bonato, “Enantioselective analysis of primaquine and its metabolite carboxyprimaquine by capillary electrophoresis”, Electrophoresis 25, 2848 (2004). doi: 10.1002/elps.200405961 34. S. Kristensen, “Photoreactivity of biologically active compounds. XVII. Influence of solvent interactions on spectroscopic properties and photostability of primaquine”, Pharmazie 60, 426 (2005). 35. S. Bhoopathy, B. Xin, S.E. Unger and H.T. Karnes, “A novel incubation direct injection LC/MS/MS technique for in vitro drug metabolism screening studies involving the CYP 2D6 and the CYP 3A4 isozymes”, J. Pharm. Biomed. Anal. 37, 739 (2005). doi: 10.1016/j. jpba.2004.08.043 36. V.G. Dongre, P.P. Karmuse, M.M. Nimbalkar, D. Singh and A. Kumar, “Application of GC-EI-MS for the identification and investigation of positional isomer in pri-. maquine, an antimalarial drug”, J. Pharm. Biomed. Anal. 39, 111 (2005). doi: 10.1016/j.jpba.2005.03.019 37. F. Zsila and G. Gedeon, “Binding of anti-prion agents to glycosaminoglycans: evidence from electronic absorption and circular dichroism spectroscopy”, Biochem. Biophys. Res. Commun. 346, 1267 (2006). doi: 10.1016/j. bbrc.2006.06.033 38. M.A. La-Scalea, C.M.S. Menezes, G.C. Matsutami, M.C. Polli, S.H.P. Serrano and E.I. Ferreira, “Molecular modeling of the voltammetric oxidation at a glassy carbon electrode of the antimalarial drug primaquine and its prodrugs succinylprimaquine and maleylprimaquine”, Electrochim. Acta 51, 5103 (2006). doi: 10.1016/j.electacta.2006.03.085 39. B. Avula, L.M. Tripathi, S.I. Khan, B.L. Tekwani, D. Nanayakkara, W. Gul, M.A. Elsohly and I.A. Khan, “LC method for the determination of NPC1161, primaquine and their metabolites in various biological systems”, Chromatographia 64, 429 (2006). doi: 10.1365/s10337006-0049-x 40. I. Brondz, D. Ekeberg, D.S. Bell, A.R. Annino, J.A. Hustad, R. Svendsen, V. Vlachos, P. Oakley, G.J. Langley, T. Mohini, C.G. Amaury and F. Mikhalitsyn, “Nature of the main contaminant in the drug primaquine diphosphate: SFC and SFC–MS methods of analysis”, J. Pharm. Biomed. Anal. 43, 937 (2007). doi: 10.1016/j. jpba.2006.09.017 41. V.G. Dongre, P.P. Karmuse, P.P. Rao and A. Kumar, “Development and validation of UPLC method for determination of primaquine phosphate and its impurities”, J. Pharm. Biomed. Anal. 46, 236 (2008). doi: 10.1016/j. jpba.2007.09.012 42. N. Vale, J. Matos, R. Moreira and P. Gomes, “Amino acids as selective acylating agents: regiosselective N1-acylation of imidazolidin-4-one derivatives of the antimalarial drug primaquine”, Tetrahedron 64, 11144 (2008). doi: 10.1016/j.tet.2008.09.058 43. S. Vangapandu, S. Sachedva, M. Jain, S. Singh, P.P. Singh, L.C. Kaul and R. Jain, “8-Quinolinamines and their prodrug conjugates as potent blood-Schizontocidal antimalarial agents”, Bioorg. Med. Chem. 11, 4557 (2003). doi: 10.1016/j.bmc.2003.07.003 44. M. Jain, S. Vangapandu, S. Sachdeva, S. Singh, P.P. Singh, G.B. Jena, K. Tikoo, P. Ramarao, C.L. Kaul and R. Jain, “Discovery of a bulky 2-tert-butyl group containing primaquine analogue that exhibits potent bloodschizontocidal antimalarial activities and complete elimination of methemoglobin toxicity”, J. Med. Chem. 47, 285 (2004). doi: 10.1021/jm0304562 45. I. Slepukhina, T. Duelcks, H.M. Schiebel and D. Gabel, “Fragmentation of B12H11S-R(2−) in electrospray mass spectrometry, J. Organomet. Chem. 690, 2796 (2005). doi: 10.1016/j.jorganchem.2005.01.040 46. http://www.thermofinnigan.com 47. W.F. Smyth, J.C. Leslie, S. McClean, B. Hannigan, H.P. McKenna, B. Doherty, C. Joyce and E. O’Kane, “The.
(13) N. Vale et al., Eur. J. Mass Spectrom. 15, 627–640 (2009). characterisation of selected antidepressant drugs using electrospray ionisation with ion trap mass spectrometry and with quadrupole time-of-flight mass spectrometry and their determination by high-performance liquid chromatography/electrospray ionisation tandem mass spectrometry”, Rapid Commun. Mass Spectrom. 20, 1637 (2006). doi: 10.1002/rcm.2485 48. K.X. Wan, T. Shibue and M.L. Gross, “Non-covalent complexes between DNA-binding drugs and doublestranded oligodeoxynucleotides: a study by ESI ion-trap mass spectrometry”, J. Am. Chem. Soc. 122, 300 (2000). doi: 10.1021/ja990684e 49. A.M. Clark, C.D. Hufford, R.K. Puri and J.D. McChesney, “Production of a novel dimeric metabolite of primaquine by Streptomyces rimosus”, App. Environ. Microbiol. 47, 540 (1984). 50. C.D. Hufford, A.M. Clark and J.D. McChesney, “Stereochemistry of dimeric microbial metabolites of primaquine”, J. Org. Chem. 49, 2822 (1984). doi: 10.1021/ jo00189a039 51. M. Sugumaran and E. Nelson, “Model sclerotization studies. 4. Generation of N-acetylmethionyl catechol adducts during tyrosinase-catalyzed oxidation of catechols in the presence of N-acetylmethionine”, Arch. Insect. Biochem. Physiol. 38, 44 (1998). doi: 10.1002/ (SICI)1520-6327(1998)38:1<44::AID-ARCH5>3.0.CO;2-V 52. D. Pietraforte and M. Minetti, “One-electron oxidation pathway of peroxynitrite decomposition in human blood plasma: evidence for the formation of protein tryptophan-centred radicals”, Biochem. J. 321, 743 (1997). 53. W. Vost, “Oxidation of methionyl residues in proteins: Tools, targets, and reversal”, Free Radical Biol. Med. 18, 93 (1995). doi: 10.1016/0891-5849(94)00158-G 54. H. Sun, J. Gao, D.A. Ferrington, H. Biesiada, T.D. Williams and T.C. Squaier, “Repair of oxidized calmodulin by methionine sulfoxide reductase restores ability to activate the plasma membrane Ca-ATPase”, Biochemistry 38, 105 (1999). doi: 10.1021/bi981295k 55. J. Moskovitz, H. Weissbach and N. Brot, “Cloning the expression of a mammalian gene involved in the reduction of methionine sulfoxide residues in proteins”, Proc. Natl. Acad. Sci. 93, 2095 (1996). doi: 10.1073/ pnas.93.5.2095 56. R.L. Levine, L. Mosoni, B.S. Berlett and E.R. Stadtman, “Methionine residues as endogenous antioxidants in proteins”, Proc. Natl. Acad. Sci. 93, 15036 (1996). doi: 10.1073/ pnas.93.26.15036 57. H. Liu, G. Gaza-Bulseco and L. Zhou, “Mass spectrometry analysis of photo-induced methionine oxidation of a recombinant human monoclonal antibody”, J. Am. Soc. Mass Spectrom., in press (2008). 58. M.M. Cordero, J.J. Houser and C. Wesdemiotis, “The neutral products formed during backbone fragmentations of protonated peptides in tandem mass spectrometry”, Anal. Chem. 65, 1594 (1993). doi: 10.1021/ ac00059a019. 639. 59. B. Paisz and S. Suhai, ”Combined quantum chemical and. RRKM modeling of the main fragmentation pathways of protonated GGG. I. Cis-trans isomerization around protonated amide bonds”, Rapid Commun. Mass Spectrom. 15, 2307 (2001). doi: 10.1002/rcm.507 60. B. Paisz and S. Suhai, ”Combined quantum chemical and RRKM modeling of the main fragmentation pathways of protonated GGG. II. Formation of b2, y1, and y2 ions”, Rapid Commun. Mass Spectrom. 16, 375 (2002). doi: 10.1002/rcm.586 61. B. Paisz, S. Suhai and A.G. Harrison, “Experimental and theoretical investigation of the main fragmentation pathways of protonated H–Gly–Gly–Sar–OH and H–Gly–Sar– Sar–OH, J. Am. Soc. Mass Spectrom. 14, 1454 (2003). doi: 10.1016/j.jasms.2003.07.001 62. B. Paisz and S. Suhai, “Towards understanding the tandem mass spectra of protonated oligo peptides. 1: mechanism of amide bond cleavage”, J. Am. Soc. Mass Spectrom. 15, 103 (2004). doi: 10.1016/j. jasms.2003.09.010 63. L.L. Smith, K.A. Herrmann and V.H. Wysocki, “Investigation of gas phase ion structure for prolinecontaining b2 ion”, J. Am. Soc. Mass Spectrom. 17, 20 (2006). doi: 10.1016/j.jasms.2005.06.016 64. J. Laskin, T.H. Bailey and J.H. Futrell, “Mechanisms of peptide fragmentation from time- and energy-resolved surface-induced dissociation studies: Dissociation of angiotensin analogs”, Int. J. Mass Spectrom. 249/250, 462 (2007). doi: 10.1016/j.ijms.2005.11.007 65. V. Ramesh, P. Nagi Reddy, R. Srinivas, G. Srinivasulu and A.C. Kunwar, “Differentiation of three pairs of positional isomers of hybrid peptides with repeats of phenylalanine-b 3-h-valine/b 3-h-valine-phenylalanine by electrospray ionization tandem mass spectrometry”, Rapid Commun. Mass Spectrom. 21, 1401 (2007). doi: 10.1002/ rcm.2975 66. B. Balta, V. Avyente and C. Lifshitz, “Elimination of water from the carboxyl group of GlyGlyH+”, J. Am. Soc. Mass Spectrom. 14, 1192 (2003). doi: 10.1016/S10440305(03)00479-3 67. C. Hansch and A. Leo, Substituent Constants for Correlations Analysis in Chemistry and Biology. Wiley Interscience, New York, USA, p. 527 (1979). 68. R.A. Jockusch, P.D. Schnier, W.D. Price, E.F. Strittmatter, P.A. Demirev and E.R. Williams, “Effects of charge state on fragmentation pathways, dynamics, and activation energies of ubiquitin ions measured by blackbody infrared radiative dissociation”, Anal. Chem. 69, 1119 (1997). doi: 10.1021/ac960804q 69. Q. Zhang, V.H. Wysocki, P.Y. Scaraffia and M.A. Wells, “Fragmentation pathway for glutamine identification: loss of 73 Da from dimethylformamidine glutamine isobutyl ester”, J. Am. Soc. Mass Spectrom. 16, 1192 (2005). doi: 10.1016/j.jasms.2005.03.052 70. H. Li and G. Yuan, “Electrospray ionization mass spectral characteristics and fragmentation mechanisms of.
(14) 640. ESI-MS Characterization of Novel Primaquine Peptidomimetic Derivatives. angiotensin II and its analogues”, Int. J. Mass Spectrom. 252, 54 (2006). doi: 10.1016/j.ijms.2006.02.016 71. Z.T. Zhu, Y.M. Li, Y.T. Guo, M. Sun and Y.F. Zhao, “Preliminary ESI-MS and MALDI-TOF analysis on phosphoryllated tetrapeptides with Xaa–Pro motif”, Phosphorus Sulfur 182, 825 (2007). doi: 10.1080/10426500601059482 72. C. Santos, M.L. Mateus, A.P. dos Santos, R. Moreira, E. de Oliveira and P. Gomes, “Cyclization-activated prodrugs. Synthesis, reactivity and toxicity of dipeptide esters of paracetamol”, Bioorg. Med. Chem. Lett. 15, 1595 (2005). doi: 10.1016/j.bmcl.2005.01.065 73. H. Bundgaard and M. Johansen, “Prodrugs as drug delivery systems IV: N-Mannich bases as potential. novel prodrug for amides, ureides, amines, and other NH-acidic compounds”, J. Pharm. Sci. 69, 44 (1980). doi: 10.1002/jps.2600690112 74. G.J. Rasmussen and H. Bundgaard, “Prodrug of peptides. 10. Protection of di- and tripeptides against aminopeptidase by formation of bioreversible 4-imidazolidinone derivatives”, Int. J. Pharm. 71, 45 (1991). doi: 10.1016/0378-5173(91)90066-W 75. G.J. Rasmussen and H. Bundgaard, “Prodrug of peptides. 11. Imidazolidinone prodrug derivatives of enkephalins to prevent aminopeptidase–catalyzed metabolism in plasma and absorptive mucosae”, Int. J. Pharm. 76, 113 (1991). doi: 10.1016/03785173(91)90349-S.
(15)
Imagem
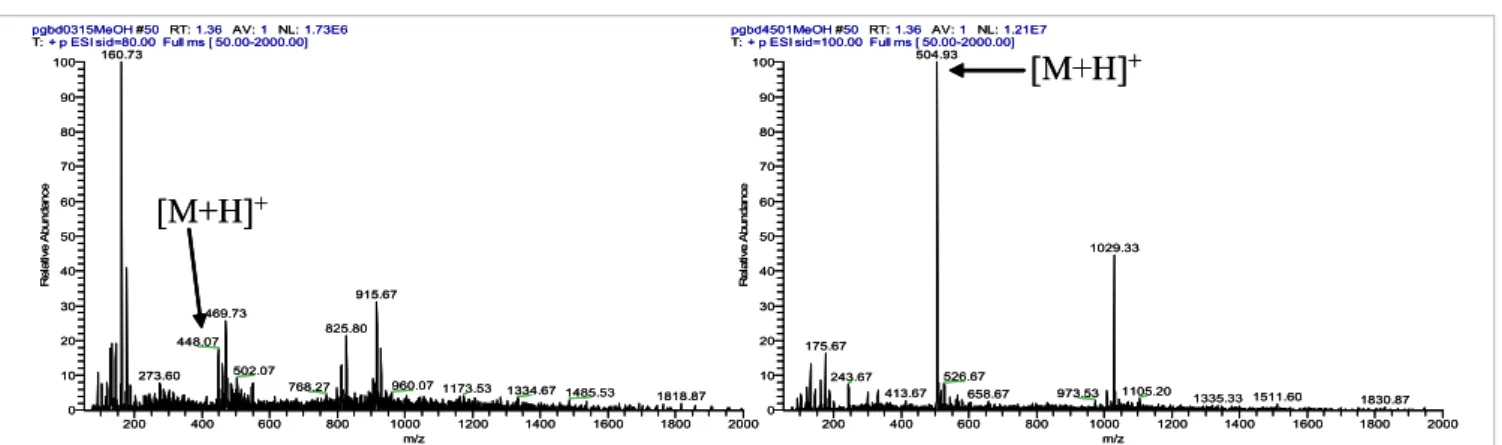
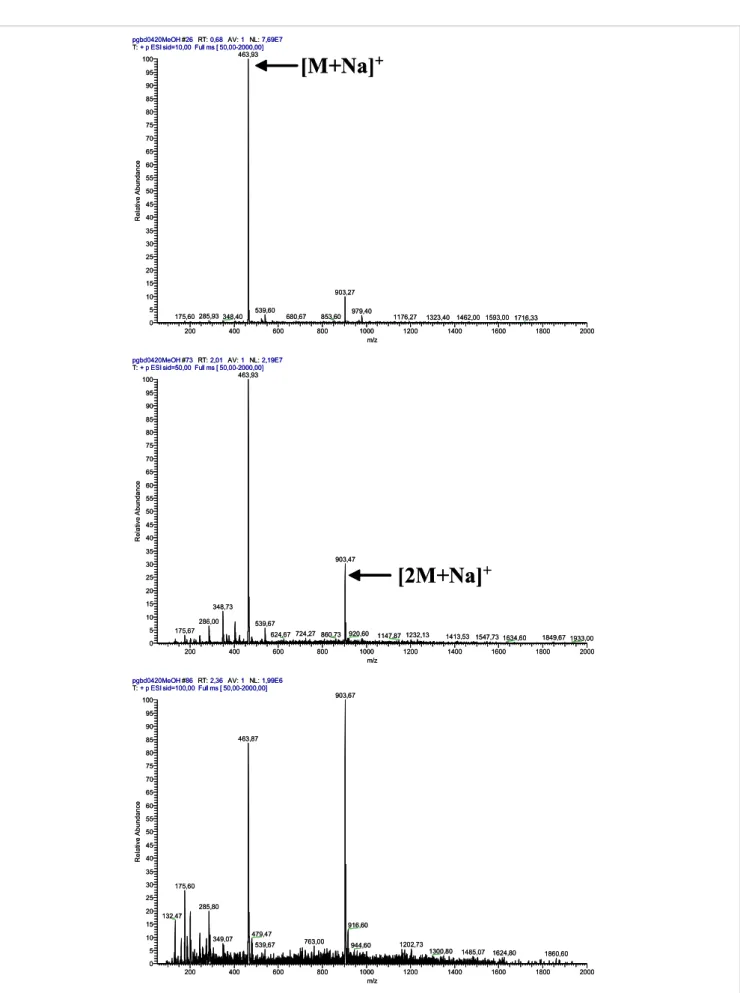
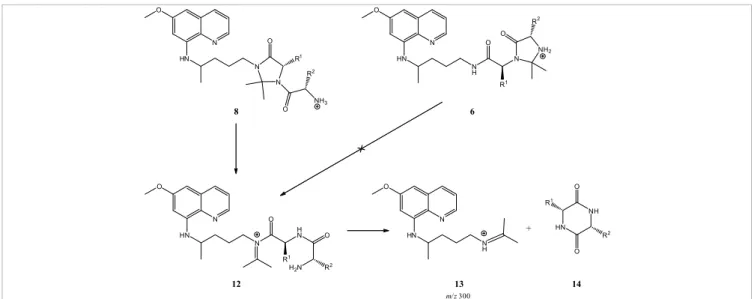

Documentos relacionados
75 equipe composta por assistente social, psicóloga, pedagogo, eu acho que isso cumpre uma função de tentar ajudar esse aluno pra problemas que não sejam referentes a ensino
Este artigo apresenta uma análise sobre as práticas escolares de Educação Moral e Cívica (EMC) nas escolas do Oeste de Santa Catarina, durante o período de 1969 a 1993, a partir
The overexpression of content (Amylase, Carboxypeptidase and Trypsinogen), matrix (ZG16p) and membrane (GP2) proteins in untreated AR42J cells and in
Vão para uma mesa (não consigo ver e não oiço o que dizem). Sentam-se numa mesa. Vejo que está a ajudar uma delas a arrumar o caderno de Matemática enquanto a outra procura
In this short report, we present the first investigation of the gas-phase chemistry fragmentation reactions of seriniquinone in electrospray ionization tandem mass
ULTRASONIC ENERGY AS A TOOL IN THE SAMPLE TREATMENT FOR POLYMERS CHARACTERIZATION THROUGH MATRIX-ASSISTED LASER DESORPTION IONIZATION TIME-OF-FLIGHT MASS
do mestrando e de acordo com os dados disponíveis é possível afirmar que o arranque do sistema SAP, não estava em causa aquando desta data, uma vez que para além da direcção de
Destacam-se como objetivos do estudo identificar os fatores de risco, intrínsecos e extrínsecos, que contribuem para a ocorrência de quedas de idosos no domicílio