Regulation of body weight in small rodents : a trade-off between starvation and predation?
Texto
(2) . .
(3) This study was funded by Fundação para a Ciência e a Tecnologia (FCT) through a PhD fellowship – SFRH/BD/47333/2008. . This thesis should be cited as follows: . Monarca, R.I., 2015. Regulation of body weight in small rodents: a trade‐off between starvation and predation?. Ph.D. Thesis. University of Lisbon. Portugal. . . .
(4) . .
(5) PRELIMINARY NOTES According to the Post‐graduate Studies Regulation (Diário da República, 2ª série, nº 57, 23 March 2015) this dissertation includes papers published or submitted for publication and the candidate, as co‐author. As doctoral candidate I was responsible for the scientific planning, sampling design, data collection, statistical analyses and writing of all manuscripts. The advisors of the thesis were deeply involved in the conceptual framework and in all the stages of the studies. Papers format was made uniform to improve text flow. Lisboa, Junho de 2015 Rita I. Monarca . . NOTA PRÉVIA Na elaboração desta dissertação foram usados artigos publicados, ou submetidos para publicação, em revistas científicas indexadas. De acordo com o previsto no Regulamento de Estudos Pós‐ Graduados da Universidade de Lisboa, publicado no Diário da República, 2.ª série, n.º 57, de 23 de Março de 2015, a candidata esclarece que participou na concepção, obtenção dos dados, análise e discussão dos resultados de todos os trabalhos, bem como na redacção dos respectivos manuscritos. A dissertação, por ser uma compilação de publicações internacionais, está redigida em Inglês. A formatação do texto foi uniformizada de modo a tornar a leitura mais fluída. Lisboa, Junho de 2015 Rita I. Monarca . . . .
(6) . .
(7) Index ACKNOWLEDGMENTS .................................................................................................... I . RESUMO ...................................................................................................................... III . ABSTRACT .................................................................................................................... IX . CHAPTER 1 .................................................................................................................... 1 GENERAL INTRODUCTION 1.1. SCIENTIFIC FRAMEWORK ............................................................................................. 3 1.1.1. ENERGY – GENERAL PRINCIPLES ....................................................................................... 3 1.1.2. INTERACTION BETWEEN ENERGY COMPONENTS ................................................................... 7 MODELS EXPLAINING ENERGY HOMEOSTASIS ............................................................................. 7 1.1.3. SIGNALLING OF ENERGY BALANCE ................................................................................... 11 1.1.4. FACTORS INFLUENCING ENERGY BALANCE ......................................................................... 13 PREDATION ....................................................................................................................... 14 LIMITED FOOD RESOURCES ‐ STARVATION ............................................................................... 16 1.1.5. DYSREGULATION OF ENERGY BALANCE – OBESITY .............................................................. 17 1.2. AIMS AND APPROACH .............................................................................................. 23 1.3. THESIS ORGANISATION ............................................................................................. 25 1.4. REFERENCES .......................................................................................................... 26 . CHAPTER 2 .................................................................................................................. 35 PREDATION RISK AND STARVATION 2.1 ABSTRACT .............................................................................................................. 37 .
(8) 2.2. INTRODUCTION ...................................................................................................... 38 2.3. MATERIAL AND METHODS ........................................................................................ 40 2.4. RESULTS ............................................................................................................... 49 2.5. DISCUSSION .......................................................................................................... 59 2.6. CONCLUSIONS ........................................................................................................ 66 2.7. ACKNOWLEDGEMENTS ............................................................................................. 66 2.8. REFERENCES .......................................................................................................... 66 . CHAPTER 3 .................................................................................................................. 71 GROWTH AND DEVELOPMENT 3.1. ABSTRACT ............................................................................................................. 73 3.2. INTRODUCTION ...................................................................................................... 74 3.3. METHODS ............................................................................................................. 76 3.4. RESULTS ............................................................................................................... 80 3.5. DISCUSSION .......................................................................................................... 86 3.6. CONCLUSION ......................................................................................................... 92 3.7. REFERENCES .......................................................................................................... 93 . CHAPTER 4 .................................................................................................................. 99 PHYSICAL ACTIVITY AND BEHAVIOUR 4.1. ABSTRACT ............................................................................................................101 4.2. INTRODUCTION ............................................................................................................ 102 4.3. METHODS .................................................................................................................. 103 4.4. RESULTS ..................................................................................................................... 108 4.5. DISCUSSION ................................................................................................................ 115 .
(9) 4.6. REFERENCES ................................................................................................................ 118 . CHAPTER 5 ................................................................................................................ 123 GENERAL DISCUSSION 1) DO ANIMALS UNDER PERCEIVED RISK OF PREDATION ADJUST THEIR BODY WEIGHT? ...................... 126 2) WHICH PHYSIOLOGICAL AND BEHAVIOUR FACTORS ARE CHANGED TO PRODUCE SUCH ADJUSTMENTS? ..... 127 . 3) WHAT SIGNAL THE ENERGY BALANCE AND IMBALANCE TO PRODUCE SUCH ADJUSTMENTS? ............ 130 IMPLICATIONS AND FUTURE DIRECTIONS ..................................................................................... 131 REFERENCES ......................................................................................................................... 134 . . . .
(10) .
(11) ACKNOWLEDGMENTS . Taking the final steps of long journey, there are several people who crossed my way and whom I am greatly indebted. At institutional level, I would like to thank “Fundação para a Ciência e Tecnologia” for funding my work through a PhD grant. To my supervisors for accepting the challenge of accompanying along this journey. To Professor Maria da Luz Mathias, who many years ago put some faith in me by accepting me into the “small mammal group” and who across the years have been getting used to my simple ways of seeing the world, sometimes called minimalistic. My sincere gratitude to Professor John Speakman, for all his guidance, helping cross several bumps along the way and for making all these energetics related topics much more familiar. In Lisbon, to my colleagues Joaquim, Sofia, Ana Cerveira, Cristiane, Isabel, Flávio, Margarida and Ana Quina, for their constant help, words of support and scientific input. Ana Cerveira, Joaquim and Sofia, many thanks for the labour hours helping me on the field work and maintaining the animals when I was away. I hope that in a near future our out of the box ideas for research projects will see the light of day. Patricia Napoleão, thank you for helping me with the first steps into the biochemistry lab. In Aberdeen, to the energetics research group, Aqeel, Mia, Rachel, Madu, Quinn, Sharon, David, Catherine, and Lobke, many thanks for your support. A special word for Lobke, for all the help on the constant attempts of getting the study up and running. In Beijing, to the lab colleagues, Teresa, Li‐Li, Deng Bao, Yanchao, Xinyu, Mengqin, Chaoqun, Guanlin, I would like to thank you all for the way I was welcome into the group, you were never refused me any help dealing with lab issues or every day issues of a non‐ speaker of the local language. To Professor De‐Hua Wang, thank you very much for . I .
(12) proving part of the equipment to perform the experiments. To Teresa and Panqian, thanks for the really fun moments we shared during our city adventures. A special thanks to Paula Lopes for the amazing help on putting together the first foundations of this project, and for every now and then quick word of advice. To worldwide spread but always close friends Andreia, Sofia, Guida, Hugo, Paulo, Raul e Inês(es), feels good to know I can count on you. To my mother who always supported my choices and to my core family thank you for the encouragement along this journey. Tiago, thank you so much for all the encouragement, support, patience and love, to make it through the times apart and always be there for me, I hope that finally we can spend more time together. . . II .
(13) RESUMO Os mecanismos envolvidos na regulação do peso do corpo, assim como as pressões evolutivas que controlam estes mecanismos, têm sido largamente discutidos. Diversas teorias têm proposto uma panóplia de modelos explicativos, que incluem diversas componentes, como sobrevivência em períodos de escassez de recursos, sucesso reprodutor, alterações de comportamento ou ausência de co‐adaptação às actuais dietas. No entanto, o funcionamento destes mecanismos e a interacção dos factores que os influenciam permanece em grande parte desconhecida. . A desregulação do balanço energético pode originar situações de excesso de peso e em casos extremos conduzir à obesidade. Durante o século XX, a incidência de obesidade aumentou nas sociedades ocidentais de forma epidémica. Na base desta desregulação existe uma forte componente genética, mas também uma forte influência ambiental. A capacidade de armazenar energia na forma de gordura, pode representar uma vantagem adaptativa visto conferir um grau de protecção contra períodos em que os recursos alimentares são escassos e que podem incorrer em eventos de fome. . O modelo clássico de regulação de peso propõe que o peso corporal seja pré‐determinado internamente, dependendo exclusivamente de factores individuais. Sempre que o peso do corpo varia acima ou a abaixo deste valor pré‐estabelecido são desencadeados mecanismos que actuam de modo a restabelecer o peso corporal inicial. Recentemente, outros modelos têm surgido, nomeadamente o modelo de duplo ponto de intervenção, que propõe não a existência de um valor pré‐determinado, mas a existência de um III .
(14) intervalo, com um limite inferior e um limite superior, entre os quais o peso pode variar livremente. Quando o peso atinge o limite superior, ou o limite inferior do intervalo, são activados mecanismos que restabelecem o peso do corpo para o intervalo pré‐ estabelecido. . Tem sido demonstrado que os pequenos mamíferos possuem mecanismos de regulação do peso do corpo muito eficientes. O acesso permanente a alimento ou a modificação da alimentação para dietas muito ricas em gordura raramente resultam em aumentos de peso que possam ser classificados como obesidade. No entanto, os factores que condicionam estes mecanismos são ainda desconhecidos. O risco de predação, assim como o risco de fome têm sido indicados como dois factores que condicionam os limites de variação do peso do corpo, mantendo‐o dentro do intervalo pré‐determinado. . Neste trabalho, utilizando pequenos mamíferos como modelos de estudo, analisou‐se a possível existência de um equilíbrio entre o risco de predação e o risco de fome, testando‐ se experimentalmente a hipótese segundo a qual o desaparecimento da pressão de predação conduzirá à proliferação do fenótipo associado à acumulação de gordura. Segundo esta hipótese, os genes responsáveis pela eficiente acumulação de reservas de gordura terão sido mantidos no pool genético por um fenómeno de deriva genética, em humanos, depois da eliminação do risco de fome e predação, das populações ancestrais, devido ao desenvolvimento da agricultura e armas, estes genes deveriam ter sido eliminados, por terem perdido o seu valor adaptativo. . Para a avaliação dos efeitos do risco de predação, pequenos mamíferos foram submetidos a estímulos sonoros que simulam a presença de um predador, e as suas respostas IV .
(15) fisiológicas e comportamentais foram analisadas. Esta metodologia foi aplicada a duas espécies de pequenos roedores, o rato‐do‐campo Apodemus sylvaticus e à estirpe C57BL/6 de ratinhos de laboratório. Pretendeu‐se investigar se o risco de predação afecta o peso do corpo dos animais e de que modo se processa este efeito, nomeadamente em termos de consumo de comida, alterações de padrões de comportamento, metabolismo, termorregulação e actividade física. Pretendeu‐se também analisar se os mecanismos envolvidos na regulação do balanço energético são sinalizados através de mensagens hormonais que actuam a nível do sistema nervoso central. . Os resultados obtidos mostram uma significativa influência do risco de predação sobre o peso do corpo, por outras palavras, os animais submetidos a um tratamento que simula a presença de um predador reduziram o seu peso corporal ou a taxa de ganho de peso quando submetidos a dietas com elevado teor de gordura. Esta alteração é feita através da variação tanto da quantidade de energia ingerida, como da quantidade de energia gasta, processos que podem funcionar de forma independente ou em simultâneo. A redução da quantidade de energia ingerida baseia‐se principalmente na diminuição da quantidade de comida consumida e não na alteração das taxas de digestibilidade e absorção. O aumento da energia despendida ocorre através da alteração dos padrões de atividade física e comportamento, ou seja, os animais favorecem comportamentos que aumentam a actividade física e que são mais dispendiosos do ponto de vista energético, como moveram‐se dentro da caixa, em oposição a manterem‐se imóveis ou em descanso como método de poupança de energia. A taxa de metabolismo basal, que muitas vezes é associada a poupança de energia, em animais que estão adaptados a ambientes extremos . V .
(16) como desertos ou ambientes subterrâneos, não é alterada em consequência do risco de predação. . A exposição estocástica a períodos de fome induziu reduções acentuadas do peso do corpo, que foram recuperadas assim que a disponibilidade de comida foi restabelecida. A compensação do peso perdido foi feita através do aumento da comida ingerida, e da alteração de comportamento, sendo adoptados comportamentos que reduzem o gasto energético. Ao contrário do previsto, após a exposição a vários períodos de fome, os animais não sobrecompensaram a massa corporal perdida, ou seja, o peso corporal é restabelecido para valores semelhantes ao período de referência, anterior aos eventos de fome, não sendo estabelecido um novo limite de regulação. Segundo o previsto pelo modelo de duplo ponto de intervenção, esperava‐se que o aumento da frequência de períodos de fome reestabelecesse o nível mínimo de reservas para um limite superior, ao de referência, de modo a assegurar a sobrevivência em próximos eventos de escassez. . Os dados revelaram também algumas diferenças no que se refere à resposta de machos e fêmeas, tendo as fêmeas exibido uma resposta evidente ao elevado risco de predação, principalmente quando expostas a dietas com alto teor de gordura. A resposta à exposição a uma dieta rica em gordura, após um período inicial de dieta pouco rica em gordura, também é diferente entre machos e fêmeas, tendo as fêmeas demonstrado maior resistência ao aumento de peso que os machos. Estas diferenças poderão estar relacionadas com a influência do tamanho do corpo em questões de dominância e agressividade, e com o investimento na reprodução. . VI .
(17) Os níveis de corticosterona foram alterados em resposta ao aumento do risco de predação, mas os níveis de leptina circulante são explicados principalmente pelas reservas de gordura acumuladas. No entanto, os animais submetidos a períodos de fome registaram elevados níveis de leptina circulante, o que é uma resposta inesperada face ao observado em humanos após dietas de redução de peso, em que o abandono das dietas para perda de peso é atribuído à manutenção de baixos níveis de leptina, após a dieta. . Estes resultados suportam a importância do papel da pressão de predação sobre a regulação do peso do corpo, e demonstram que estas alterações podem ser alcançadas através do recurso a uma combinação de processos fisiológicos e comportamentais, que resultam em redução do consumo de energia ou aumento do seu gasto. . A investigação dos mecanismos que regulam as variações de peso, nomeadamente a sua componente ambiental é fundamental para a compreensão de disfunções como a obesidade e doenças associadas, nomeadamente na forma como vários fenótipos são expressos sob diferentes condições ambientais. No entanto, a conhecimento da base molecular e genética, destes mecanismos, é essencial para o desenvolvimento de novos medicamentos e formas de terapia que permitam prevenir e controlar estas disfunções. . . Palavras‐chave: Regulação de peso; Obesidade; Risco de predação; Fome. . . . VII .
(18) . .
(19) ABSTRACT The mechanisms and evolutionary background of body weight regulation have been largely discussed in the last years. Several models were put forward to explain the energetic imbalances that lead to disorders as obesity, linking environmental and genetic factors, and their effects over body weight. Unlike humans, small wild mammals have a strong body weight regulation system, being the risk of predation one of the factors suggested to explain the non‐prevalence of overweight animals within natural populations. Accordingly, carrying large fat reserves influences the ability to escape predators, being the risk of predation the main factor influencing their upper limit for body weight regulation. On the other hand the risk of starving, due to carrying reduce fat storages during famine periods is likely to guide the lower boundary for body weight regulation, a trade‐off between these two pressure factors is suggested to modulate body weight in small mammals. The predications of this model were tested using wood mice (Apodemus sylvaticus) and C57BL/6 mice by experimentally manipulating the risks of starvation and predation. Physiological and behavioural responses were tested to investigate the response of mice to elevated risks of starvation and predation. Results showed that reductions in body weight, and body weight gain, can be induced by manipulating the predation risk. The variations were mostly explained by reduction of food intake, and increase in energy expenditure through alteration of physical activity and behaviour. Resting metabolic rate and thermogenic capacity were not affected. Males and females responded differently to high fat diets, females showed stronger homeostasis mechanisms. Starvation periods were compensated by overfeeding and reduction of activity during the recovery period but did not induce the increase of the fat storages above pre‐starvation. Corticosterone levels signed the perceived risks of predation and circulating leptin was correlated with the levels of fat storage. These observation strongly support the role of predation risk over the regulation of body weight, showing the influence of environmental components over the setting of body weight regulation limits. Keywords: Predation‐Starvation trade‐off; Weight regulation; Body weight; Obesity IX .
(20) . . .
(21) . . . . . CHAPTER 1 General Introduction . . .
(22) . . .
(23) Chapter 1 – General Introduction . . General Introduction . 1.1. Scientific framework 1.1.1. Energy – General principles Energy is considered a key currency for all the biological processes. Ultimately, energy is one of the most essential resource for animals life, therefore acquiring the amount of food to supply their daily need of energy is a basic goal of every animal. . The first law of thermodynamics states that energy can be transferred and transformed, but cannot be created or destroyed (Blaxter 1989). When applied to animal physiology, this process can be summarised by the following equation, all terms expressed as energy per unit of time: . Energy Intake = Energy Expenditure + Energy Storage . Energy Intake primarily refers to the chemical energy obtained through consumed food and fluids; Energy Expenditure refers to performed work, and energy lost through radiant, conductive, and convective heat, and also evaporation. Energy Storage is the rate of change in body’s macronutrient stores (Hall et al. 2012). The balance between energy intake, energy expenditure and energy storage can be referred as energy homeostasis. . The Energy Intake components are macronutrients (proteins, carbohydrates and fat) and micronutrients (vitamins, minerals and trace elements). Micronutrients are part of the diet, but their contribution to the total energy ingested is not significant. Once ingested, 3 .
(24) Chapter 1 – General Introduction . the absorption of macronutrients is variable and incomplete, typically urine accounts for 2‐3% (Grodzínski & Wunder 1975) of total energy input, faecal loss is variable, depending on the individual variance, diet quality and intestinal factors, red pandas can lose 80% of energy input through faeces (Wei et al. 2000) and grey seals about 8% (Ronald & Keiver 1984). The metabolized energy is given by the difference between the total energy content of the consumed food and the energy content of faeces and urine. The transformed macronutrient can then be used in the biological processes or be stored. . The rate of Energy Expenditure is variable across the day and during the life span. Accordingly, maintenance, growth, reproduction, lactation, running, and other processes, all require energy but the amounts of fuel may be variable. . The energy expenditure budget can be divided in three main components: i) Resting/Basal metabolism, ii) Thermal effect of food, iii) Thermo regulatory metabolism and iv) Activity metabolism. . i) Resting metabolic rate represents approximately two‐thirds of total human energy expenditure (Cunningham 1991), and 40% of small mammals total energy expenditure (reviewed by Speakman 2000). Basal metabolic rate can be defined as the minimal rate necessary to sustain basic physiological processes, as respiration, heart rate and cellular repair, measured in an inactive, post‐absorptive, non‐reproductive animal, at an environmentally neutral temperature. This standardised estimation of metabolic rate requires several criteria to be accomplished, however in many cases these assumptions are not easy to achieve (McNab 1997). . 4 .
(25) Chapter 1 – General Introduction . Basal metabolic rate requires animals to be inactive at thermoneutrality, thus is not measured on many studies, due to the difficulties of certifying that animals are post absorptive. Thus measurements of resting metabolic rate are often used. They may be slightly higher than basal metabolic rate, due to the thermal effect of food. According with Johnstone et al. (2005), about 63% of resting metabolic rate variation can be explained by the fat free mass, 2% by the individual variation of subjects, 6% by the body fat content, 2% by age, 0.5% by analytic error and 26,6% of the variation is unexplained. . ii) The Thermal effect of food also commonly referred as Diet induced thermogenesis, and specific dynamic action (review by Secor 2009), is the energy associated to the digestion and processing of food, in other words, it is the energetic cost of digesting, assimilating and transporting nutrients from ingested food to cells and tissues. It is assumed to be a fixed percentage of total energy intake, but may be affected by the meal size and composition (Jonge & Bray 1997). Although, a small part of the thermal effect of food consists of regulated heat production to dissipate excessive energy intake (Lowell & Spiegelman 2000). . iii) In homeotherms the maintenance of a stable body temperature depends upon the balance between heat production and heat loss ‐ Thermal regulation. The heat loss through the body surface is proportional to the difference between ambient temperature and surface temperature. Therefore the energy allocated to the maintenance of body temperature is highly influenced by the ambient temperature. As the temperature falls below core temperature, the heat demands for thermoregulation increases. Basal metabolic rate, thermal effect of food and physical activity, all contribute to supply this . 5 .
(26) Chapter 1 – General Introduction . energy demand. If these sources do not supply the required energy, specific extra heat is generated to maintain a stable body temperature. . Humans, mainly in developed countries, were able to modify their environmental conditions to permanently balance the ambient temperature and their heat production (Garland et al. 2011; Speakman & Keijer 2012). These conditions do not necessarily apply to wild animals, hence the energy allocated to balance the body temperature significantly increases. The production of heat necessary to increase the body temperature may require shivering or non‐shivering. The non‐shivering thermogenesis includes the heat generation using the brown adipose tissue, not involving muscular contractions. Non‐ shivering thermogenesis and brown adipose tissue are commonly the focus of studies regarding seasonal acclimatisation of small mammals, and recently also human physiology due to its possible anti‐obesity role (Cannon & Nedergaard 2004; Seale, Kajimura & Spiegelman 2009). . iv) Physical activity can be defined as the thermic effect of any bodily movement that goes beyond the basal metabolic rate (Caspersen, Powell & Christenson 1985). It comprises the energy expenditure required for skeletal muscles to produce movement, including minimal movement, physical exercise and powerful muscular work. Physical activity and exercise are not synonymous. Physical activity includes exercise as well as other activities which involve corporal movement. In humans, there is often a partition between exercise and non‐exercise activity (Levine, Schleusner & Jensen 2000; Hollowell et al. 2009). Energy costs with physical activity are correlated with body weight and resting metabolic rate (Schoeller & Jefford 2002), but is one of the most variable energy expenditure components. 6 .
(27) Chapter 1 – General Introduction . . 1.1.2. Interaction between energy components The three components of the energy equation interact through a series of processes that favours homeostasis, responding to the energy available. As so, if the energy expenditure increases, animals must increase their food intake to compensate the discrepancy or use the energy stored. Consequently, energy is stored if the food intake exceeds the energy expenditure. . The main components of the energy balance equation may vary across time, accordingly with the stage of development; typically energy storage is positive during growth and development resulting in the increase of body weight. During adult life, body weight is usually stable, and energy storage approaches zero. . . Models explaining energy homeostasis The regulation system underpinning energy input and expenditure has not been fully established. Multiple theories have been put forward to explain energy homeostasis; each model is the product of a different context and therefore they focus on different aspects of body weight regulation: the set point model is mostly based on physiological and genetic determination, whereas the settling point regulation model is built around the effects of social, nutritional environment. The dual point intervention model is suggested as an alternative model including weak physiological regulation within two boundaries . 7 .
(28) Chapter 1 – General Introduction . that may vary across individuals and accommodates both environmental and molecular‐ physiological views points. . a) Set point model (Lipostatic model) Kennedy (1953) was among the first to propose that the regulation of body fat involves an internal “lipostat” that maintains body fat hence overall weight at a predetermined level. It was then suggested that fat would produce a signal that once sensed by the brain would inform about the levels of fat. If these levels were above or below the target body fat, the brain would activate mechanisms to increase or reduce the energy expenditure and bring the fat levels to the target point (Figure 1). The discovery of leptin many years later (Zhang et al. 1994) gave strong molecular support to the model suggested by Kennedy, by exposing the presence of a hormonal signal that informs the brain about the fat status of the body, as reviewed by Friedman and Halaas (1998). Leptin is a hormone primarily produced by the adipose tissue and interacts with brain areas known to be related with energy homeostasis (Mercer & Speakman 2001; Bellinger & Bernardis 2002). . Figure 1 – Classic set‐point model of body weight regulation: Compensatory mechanisms are applied when body mass is below or above the set point of regulation. . 8 .
(29) Chapter 1 – General Introduction . . b) Settling point model One of the statements of the set‐point model is that it denies the role of environmental, behaviour and economic factors, resuming the mechanism is strictly due to physiological components. According to the settling point model (Wirtshafter & Davis 1977), the fat storage in a body can be compared to the water levels in a water reservoir, in analogy like a lake (Speakman et al. 2011) (Figure 2). . Figure 2 ‐ Body weight regulation according to the settling point model, here illustrated as a lake. Input of water is rain falling in the hill, the output of water is proportional to the depth of water in the outflow, as indicated by the size of the arrows. A ‐ As the volume of inflow rain increases, the depth of outflow raises; B ‐Rain fall represents energy input, energy expenditure is compared to the outflow; depth of outflow represents the settling point, and the energy storage is illustrated by the amount of water in the reservoir (lake) (adapted from Speakman et al. (2011)). . 9 .
(30) Chapter 1 – General Introduction . A natural equilibrium in maintained in the lake, due to the extra rain inflow, the water levels rises until the outflow equals the inflow. Regarding body weight, fat storages represent the lake, energy intake is represented by the rain and energy expenditure by the water outflow. In such system, there is little active regulation in order to predefine body weight, the interaction of several environmental factors (e.g. improved housing, food abundance) results in a dynamic equilibrium (“settling point”) dependent on the individual constitution and interaction between energy input and expenditure (Dubois et al. 2012). The settling point model denies any role for the physiological regulation of body fatness and weight. . . c) Dual intervention point model Contrarily to the suggested by the classic “lipostat” model of Kennedy (1953), the dual intervention point model argues that body weight is set within a range limited by an upper limit point and a lower limit point (Levitsky 2002; Speakman 2007). If body weight rises above the upper limit or decreases below the lower limit, the individual enables physiological mechanisms that change energy balance to maintain the body weight within the pre‐set range. Between these boundaries the physiological regulation is poor (Figure 3). The nature of the intervention points is still unclear, they might depend on a combination of genetic and environmental factors acting together. A strong evolutionary background has supported this model, the upper intervention point defined by the risk of predation and the lower intervention point established by the risk of starvation (Speakman 2007, 2008). The upper limit point of intervention is mostly supported by data . 10 .
(31) Chapter 1 – General Introduction . obtained in animal studies, given that since 2 million year ago modern humans have developed tools, weapons and strategies to avoid predation. . Figure 3 ‐ The dual intervention point model. Over time body weight varies, when the intervention points are reached physiological feedback controls that operate in order to reduce or increase the body mass. . 1.1.3. Signalling of energy balance Insulin was the first hormonal signal to be related with the regulation of energy balance. It is secreted by beta cells in the pancreas and enters in central nervous system via the circulation. Insulin is considered an “adiposity signal” that supresses food intake, and promotes lipid storage (Assimacopoulos‐Jeannet et al. 1995). The discovery of leptin, a hormone secreted by the adipose tissue, provided additional information about the signals sent to brain (Zhang et al. 1994). Both insulin and leptin have been involved in the regulation of body weight (Figure 4) (Varela & Horvath 2012), as their levels are proportional to the amount of body fat (Bagdade, Bierman & Porte 1967; Considine et al. 1996), and their delivery on the brain is directly related to their circulating levels (Schwartz et al. 1996). 11 .
(32) Chapter 1 – General Introduction . Two neuronal populations NPY/AGRP and POMC/CART, located in the arcuate area of the hypothalamus (Hahn et al. 1998), express specific neuropeptides known to be involved in energy homeostasis and related with food intake. Both leptin and insulin receptors are expressed at the NPY and POMC neurons at the hypothalamus (Cowley et al. 2001; Könner et al. 2007). NPY/AGRP are inhibited by leptin, hence activated by low levels of leptin, food intake is stimulated by fasting due to the activation of NPY. On the other hand, POMC neurons are stimulated by leptin and inhibited by NPY (Cowley et al. 2001). Insulin signalling in the brain is essential for glucose homeostasis, POMC insulin sensitive neurons may be involved in response to positive energy balance through promoting fat storage in white adipose tissue (Hill et al. 2010). . Figure 4 ‐ Leptin and insulin secreted by white adipose tissue (WAT) and the pancreas, respectively, inform the hypothalamus about the energy status of the organism, regulating several peripheral functions. Arc‐ arcuate nucleus; AgRP‐ agouti‐related protein; InsR‐ insulin receptor; LepR‐b‐ leptin receptor b; POMC‐ proopiomelanocortin; VMH‐ ventromedial hypothalamus. (adapted from Varela & Horvath (2012)). . 12 .
(33) Chapter 1 – General Introduction . . 1.1.4. Factors influencing energy balance Factors affecting the energy balance are linked to two main pillars, genetics and environment. Both factors interact at different levels, linking and affecting several components of energy expenditure and intake and the overall energetic balance, as represented in Figure 5. . Figure 5 ‐ Linkages among genetics and environmental effects over energy balance, through several co‐existent mechanisms (adapted from Speakman 2004). . Several loci have been identified as related with energy balance. Studies with identical twin suggest that 60 to 70% of body mass index variance is due to genetic differences (Allison et al. 1996; Segal et al. 2009). It has also been demonstrated that genes can influence the food intake (Rankinen & Bouchard 2006), weight gain (Bouchard et al. 1990), physical activity (Mustelin et al. 2012) and basal metabolic rate (Rønning et al. 2007). . 13 .
(34) Chapter 1 – General Introduction . The influence of environment over energy balance components are mostly due to alteration of behaviour, and its interaction with other components. Two main factors causing imbalance of energy homeostasis are the availability of food and physical activity. In humans, the constant food availability, their dense energetic content and portion size are likely to influence energy intake, whilst the required physical activity is reduced mostly by the characteristics of the neighbourhood namely existence of paths for walking and cycling, traffic and street safety (Lee et al. 2012), television viewing (Hernández et al. 1999) and also car use (Wen et al. 2006). . Several small mammal species, as mice and voles, use environmental cues, such as day length and temperature to trigger seasonal variations on their body mass and adiposity, making them attractive models for the study of body weight variation as these conditions can easily be simulated in laboratory (Bartness, Demas & Song 2002; Zhao, Chen & Wang 2010). Even though had been identified that small mammals have a strong body weight regulation (El‐bakry, Plunkett & Bartness 1999; Peacock & Speakman 2001), keeping their weights in a dynamic equilibrium (Levitsky 2002). . . Predation When focusing on small wild animals, the environmental components incorporates factors, such as the risk of being predated, that have lost adaptive value to humans since the development of defensive tools and discovery of fire, and evolution of social behaviour. . 14 .
(35) Chapter 1 – General Introduction . Predation represents an important element of the overall interaction between species and their survival demands, being a selective key force on the evolutionary and adaptive processes involved (Abrams 2000). Nevertheless, the effects of perceived risk of predation have been demonstrated to play a more important role shaping the preys traits than direct consumption (Preisser, Bolnick & Benard 2005; Creel & Christianson 2008) (Figure 6). . The risk of predation in known to affect morphology (McCollum & Leimberger 1997; Brönmark, Lakowitz & Hollander 2011), physiology (Slos & Stoks 2008) and behaviour (Abramsky et al. 2004; Verdolin 2006) including aggression, movement patterns (Sih & McCarthy 2002) and foraging (Brown et al. 1988; Vásquez 1994). Responses to predation risk, i.e. activation of defence mechanisms are usually assessed by a reduction in other fitness components such as reproduction or growth (Harvell 1990; Ylönen & Ronkainen 1994). . 15 .
(36) Chapter 1 – General Introduction . Figure 6 – Pathways of predation effect over prey population dynamics (adapted from Creel & Christianson 2008). . . Limited food resources ‐ Starvation All animals at some time during their life may face events of limited food resources that ultimately could lead to mortality. Starvation refers to the biological state wherein a postabsorptive animal, otherwise willing is unable to eat, as the result of extrinsic limitations on food resources (McCue 2010). . The frequency and duration of starvation events are highly variable, the predictability of such events is also variable, it occurs seasonally or randomly according with unpredictable . 16 .
(37) Chapter 1 – General Introduction . factors. Animals are constantly spending energy to maintain their basic functions, however they are not constantly acquiring energy, meaning that at any point they must rely on physiological processes to survive based on the energy internally stored, resulting in a disrupted energy balance during starvation periods. The ability to tolerate starvation varies across different animal groups, small birds and mammals may tolerate only one day of starvation (Mosin 1984; Cherel, Robin & Maho 1988), in contrast to fish (Olivereau & Olivereau 1997), amphibians (Grably & Piery 1981), and large mammals as polar bears (Atkinson & Ramsay 1995) that are often able to endure several months without feeding. Large energy reserves may extend the toleration to starvation, likewise small animals are more susceptible to food deprivation than larger animals. . Reduction of body mass associated with starvation requires prioritizing given the high demands of maintaining tissues with high turnover rates, which takes some species to catabolize tissues to save resources during starving periods, like the gastrointestinal track (Battley et al. 2000; Ferraris & Carey 2000). Liver and adipose tissue are equally mobilized (Merkle & Hanke 1988; Lamosova, Mácajová & Zeman 2004)but usually at slower rates. Skeletal muscles is less likely to be catabolize given its role in locomotion. Brain tissue, heart and reproductive organs are seldom catabolized during starving periods. . . 1.1.5. Dysregulation of energy balance – Obesity Obesity can be explained as a prolonged imbalance between energy intake and energy expenditure, where the food input exceeds the energy output and the difference is stored as fat. The accumulation of energy for future needs, in the form of fat reserves, can be 17 .
Imagem
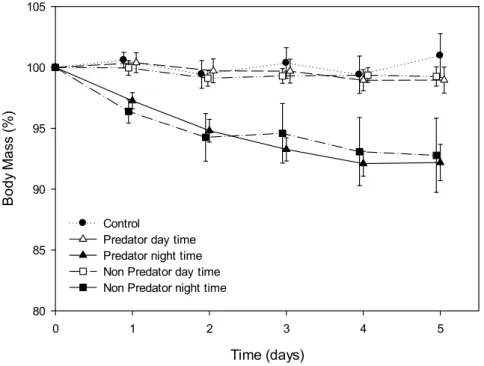
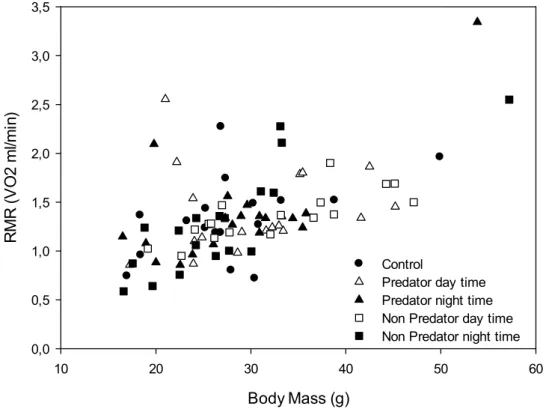
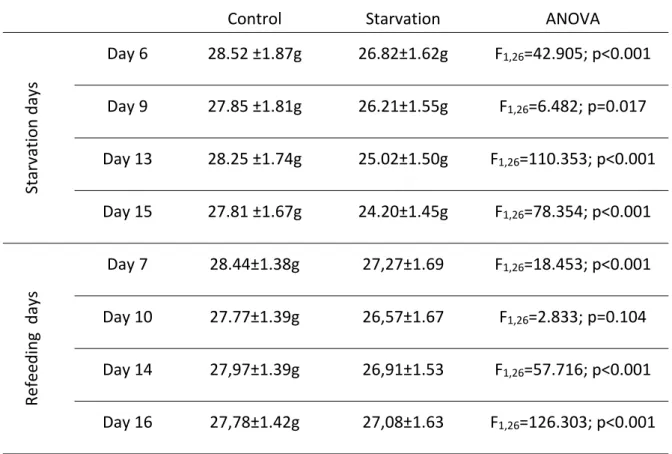
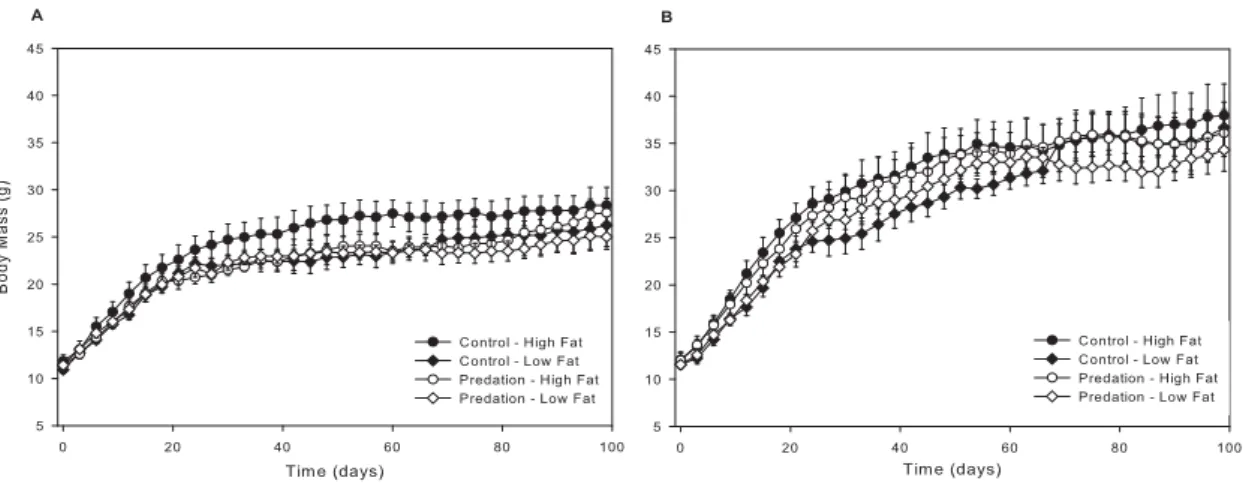
Documentos relacionados
boxes don’t have lids (no author) and don’t have any note as to what year they were assigned to the database which means that for each gem cast there needs to be
De seguida apresenta-se a colecção, e é de referir que as cores apresentadas nas ilustrações podem não corresponder na totalidade às cores escolhidas que estão referidas no
Body and cerebrum weight were measured, as well as the cholesterol and triglyceride concentrations in blood and the content of myelin, total lipids, and the concentrations of
goals of the present study were: 1) to compare the use of alternative methods to estimate body weight (weight tape and associated body-weight estimation formula) in pregnant
The relation between body weight and heart weight, between the size of the heart and the animal’s sex, the ratio between the measurements of vertical and horizontal cardiac axes,
ABSTRACT - The objective of this study was to estimate the growth parameters of body weight and body nutrient deposition in males and females of one meat- (
Objective: To compare the levels of muscle activation of the main muscle involved in gait during body weight-supported treadmill training and body weight-supported overground
Ao analisar os artigos publicados em EnANPAD’s entre os anos de 2008 a 2014, pode-se concluir que as principais correntes motivacionais que foram utilizadas