Development and characterization of ZnO piezoelectric thin film on polymeric substrates for tendon regeneration
Texto
Imagem
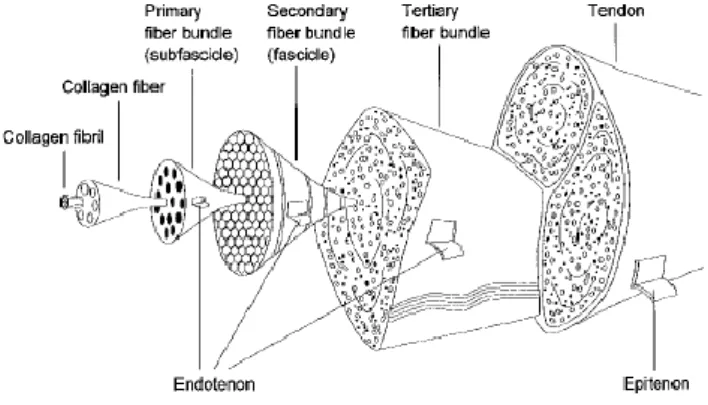
![Figure 2.2 - Stress-strain curve of normal tendon failed in tension [33]](https://thumb-eu.123doks.com/thumbv2/123dok_br/15704238.1067801/28.892.239.606.679.866/figure-stress-strain-curve-normal-tendon-failed-tension.webp)
![Figure 2.4 - Healing stages during tendon healing [47]](https://thumb-eu.123doks.com/thumbv2/123dok_br/15704238.1067801/30.892.263.628.680.902/figure-healing-stages-during-tendon-healing.webp)
![Figure 2.5 - Stages of tendon healing after midsubstance injury (adapted from [33])](https://thumb-eu.123doks.com/thumbv2/123dok_br/15704238.1067801/31.892.129.847.464.834/figure-stages-tendon-healing-midsubstance-injury-adapted.webp)
Documentos relacionados
É nesta mudança, abruptamente solicitada e muitas das vezes legislada, que nos vão impondo, neste contexto de sociedades sem emprego; a ordem para a flexibilização como
Fiquei com a sensação que este tipo de sessão não é o ideal para desenvolver num programa intergeracional de actividade física por não promover grande interacção entre idosos
The first version is similar to Antonacci procedure in the sense the MSCI USA exhibits absolute momentum if it shows a positive excess return (return less the
7) A motivação dos alunos para aprender, preparação académica dos professores e aplicabilidade das aprendizagens em profissões futuras são os principais motivos apontados
structure. The Figure 8 shows the SEM images and the optical microscopy images of samples obtained with two different temperatures 40°C and 75°C. The Figure 9 a) shows the
A análise destes fatores permite tirar conclusões sobre a forma como as economias latino-americanas têm evoluído nas últimas 2 décadas e meia e de que forma o surgimento de
Relativamente aos dados foram usados dados sísmicos (registados a distâncias telesísmicas) e geodésicos (Synthetic Aperture Radar - SAR). Quanto às técnicas foi
Figure 1 – Comparing of assessments results before and after intensive therapy, regarding the hearing-perceptive of speech analysis, nasal air emission test and hypernasality test..